Energy utilization by neurons in
the brain and spinal cord. Neurons have a high
metabolic rate and use more energy than other cells.
Although the brain accounts for 2% of total body
weight, it uses 20% of the body's total oxygen
consumption. Most energy-requiring processes in
cells use either ATP directly or energy stores
indirectly derived from ATP such as ion gradients.
Ion pumping accounts for a large part of a neuron's
energy requirement. The Na/K pump alone accounts
for 25% to 40% of a neuron's ATP utilization.
Calcium and the transport of other cations (e.g., H)
or anions (Cl or HCO3) also account for significant
energy utilization. Some pumping of Ca and H is
coupled with Na for an energy source and depletes
the Na gradient; thus, Ca and H are indirectly
coupled to ATP utilization via the Na/K pump.
Energy is required to pump ions from the cytosol to
intracellular organelles and to pump molecules
across the plasmalemma.
Transport of amino acids and other essential small
molecules across the cell membranes requires energy.
Glutamate and many other neurotransmitters are
removed from the extracellular space by active pumps
that require energy. Reduced activity
of the glutamate pump can lead to excessive
excitability and neuronal damage.
Neuronal structure and function require the
synthesis of proteins, lipids, and carbohydrates.
These substances are continually being formed,
modified, and degraded and require ATP for their
synthesis.
The transport of substances within cells also
requires energy. Most synthesis takes place in the
cell body, and an energy-dependent transport system
distributes these substances to the parts of the
neuron that require them. The enormity of the task
is apparent when one considers the length of the
axons and dendrites of a typical neuron; diffusion
is not sufficient, and active transport is required.
Energy synthesis by neurons in the brain and spinal
cord
Efficient ATP production from glucose requires
oxygen (Figure-1).
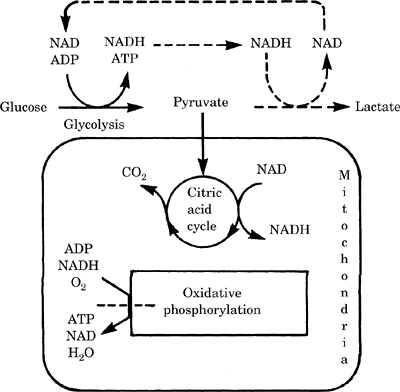
Figure-1. Energy metabolism in the brain. Dotted
lines indicate reactions that occur during ischemia.
The dotted line across the oxidative phosphorylation
reaction indicates that this reaction is blocked
during ischemia. NAD, nicotinamide adenine
dinucleotide; ADP, adenosine diphosphate; NADH,
nicotinamide adenine dinucleotide, reduced form;
ATP, adenosine triphosphate.
The major portion of energy is generated by
glycolysis (breakdown of glucose), the citric acid
cycle (a pathway that generates nicotinamide adenine
dinucleotide, reduced form [NADH], from nicotinamide
adenine dinucleotide [NAD]), and oxidative
phosphorylation (coupling of the regeneration of NAD
from NADH to the production of ATP). The
mitochondria and oxygen are critical for the
efficient production of ATP from glucose. The yield
of 1 glucose is a maximum of 38 ATP molecules
C6H12O6 & + 6O2 + 38ADP + 38Pi → 6CO2 + 6H2 +
38ATP
In an actual neuron, other synthesis pathways
use some of the energy and biochemical intermediates
so that the average yield per glucose is between 30
to 35 ATP molecules.
In the absence of oxygen, the mitochondria cannot
convert NADH to NAD and lose much of the energy of
glucose oxidation. Each molecule of glucose yields
only 2 ATP molecules. This is insufficient to meet
the energy demands of the brain. Glucose + 2ADP +
2Pi + 2NAD → 2pyruvate + 2ATP + 2NADH → 2lactate
+ 2H + 2NAD. The last step is required to regenerate
the NAD from NADH; no energy is obtained from this
step in the absence of oxygen. The H ion generated
can lead to neuronal damage under certain
circumstances.
(1) High blood glucose before hypoxia or ischemia
has been shown clinically and in animals to increase
damage. The excess H ion production could partially
explain this.
Emergency sources of energy during metabolic stress
There are two immediate sources of ATP when energy
production does not meet the cell's demand for
energy.
The enzyme adenylate kinase can convert adenosine
diphosphate (ADP) to ATP.
ADP + ADP [left harpoon over right harpoon] ATP +
AMP
When energy production recovers, this process is
reversed.
Phosphocreatine (PCr) acts as a store of high-energy
phosphate that can be rapidly converted to ATP.
Normally there are two to three times more PCr than
ATP. Nevertheless, PCr levels fall rapidly during
ischemia.
PCr + ADP + H [left harpoon over right harpoon] ATP
+ Cr
The mechanism of energy production and its reduced
usage during ischemia:
In addition to the formation of ATP from 2ADP or PCr,
anaerobic glycolysis contributes to ATP maintenance.
Anaerobic glycolysis leads to acidosis, which may be
damaging to neurons. With ischemia that lasts for
more than several minutes, ATP formation from 2ADPs
and PCr is exhausted and anaerobic glycolysis
continues only so long as glucose is available. Some
glucose is produced from the breakdown of glycogen.
Shortly after the onset of ischemia (in about 30
seconds), spontaneous neuronal activity stops and
the electroencephalogram (EEG) becomes quiet. This
reduces the neuron's metabolic rate and ATP
utilization.
The overall metabolic rate for the brain
The cerebral metabolic rate of awake young adults is
3.5 mL O2/100 g/minute or 5.5 mg glucose/100
g/minute. This rate is virtually identical in
healthy elderly persons. Children have a higher
metabolic rate, i.e., 5.2 mL O2/100 g/minute. The
reason for the higher metabolic rate in children is
unknown, but it may represent continuing growth and
development of the nervous system.
Molecular aspects of neuronal metabolism, survival
versus apoptosis
Many more neurons are formed during mammalian
development than the adult organism needs. In many
areas of the brain and spinal cord, 50% of the
neurons die as the animal matures.
The target cells of a neuron and those cells a neuron innervates, secrete trophic factors (e.g., nerve
growth factor and brain-derived neurotrophic factor)
in limited quantities. If the neuron receives enough
of these trophic factors, it survives; if not, it
dies. Because trophic factors are limited, an
average 50% of the neurons do not receive enough
trophic factors and die.
Neurons also receive trophic factors from nontarget
sources such as glia; this also promotes survival.
The combination of trophic factors from all sources
determines whether a neuron will survive or die.
The trophic factors work by binding to external
receptors on membrane-spanning proteins. When their
receptor is bound by a trophic factor, these
proteins activate intracellular signals, which in
some cases phosphorylate other intracellular
proteins and alter cellular processes leading to
neuronal survival (Figure-2).
One example of such binding is the tyrosine receptor
kinase (trk): it binds nerve growth
factor, brain-derived neurotrophic factor, or other
neurotrophins, dimerizes (combines two identical
molecules), and then becomes active. It adds a
phosphate group onto the amino acid tyrosine of
certain proteins. This addition alters the activity
of these proteins and inhibits cell death pathways.
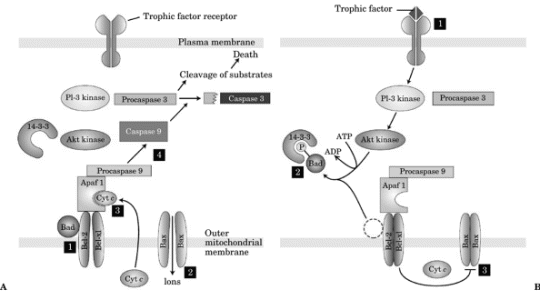
Figure-2. Trophic factors and apoptosis. A:
Absence of trophic factor: caspase activation. B:
Presence of trophic factor: inhibition of caspase
activation. ATP, adenosine triphosphate; ADP,
adenosine diphosphate.
If a cell does not receive enough trophic factor,
certain proteins are not phosphorylated and a
programmed cell death pathway is activated. This
process is called apoptosis and causes cells to die
in a way that does not cause inflammation. In the
absence of trophic factor induced phosphorylation,
a molecule called apoptosis activating factor 1
(Apaf-1) is activated, leading to the proteolysis of
inactive procaspases to active caspases, which in
turn splits other proteins and signals the cell to
undergo programmed cell death (apoptosis).
During and after ischemia, hypoxia Hypoxia, or other
injury, neurons can die by one of at least two
pathways, necrosis or apoptosis.
Necrosis is caused by severe injury that causes
neurons to swell and burst apart. In addition to
causing the death of that neuron, inflammation and
further cell death occur in that region of the
brain.
If the injury is less severe and the neuron can
recover its ability to make ATP, the neuron can
activate the apoptosis pathway and die in a manner
similar to the death of excess neurons during
development. The advantage of this apoptosis is that
the neuron does not break apart and the neurons
around it are preserved, resulting in less overall
brain damage.
Apoptosis is triggered by ion flux into the
mitochondria through an ion channel formed from the
binding of two bax protein molecules together. The
ion flux leads to the release of cytochrome c, which
binds to Apaf-1, activates it, and initiates the
caspase cascade of apoptosis (Figure-2).
|