| Postoperative
Complications |
Respiratory Care|
Cardiovascular Therapy|
Fluid Management| Nutrition in Critical Patients
|Traumatic Brain Injury-Stroke-Brain Death
|

The goals of nutritional support
in patients who are critically ill are to provide
protein and caloric replacement while attenuating a
negative nitrogen balance. It is estimated that 50%
of hospitalized patients and 60% to 80% of patients
in the intensive care unit (ICU) are malnourished.
Delays in the initiation of nutritional support may
result in muscle and gastrointestinal (GI) atrophy,
inability to be weaned from ventilatory support,
heart failure, impaired immunity, and an increase in
the incidence of sepsis, length of hospital stay,
and morbidity and mortality.
There is no doubt that effective nutritional support
coupled with appropriate monitoring improves patient
outcome. Since the early 1980s, the importance of
nutritional support in patients who have severe
neurologic injury has been appreciated. Recent
studies have also documented the same nutritional
and metabolic requirements in patients who have
acute ischemic injury and other critical neurologic
illnesses. Compelling evidence now exists that
strict glycemic control in critically ill patients
reduces morbidity and mortality.
I. Pathophysiology
The effects of elective
operations, trauma, and critical illness activate
the neural and endocrine systems. The resultant
increase in sympathetic outflow leads to lipolysis,
proteolysis, and decreased glucose uptake owing to
the antagonism of insulin by growth hormone and
epinephrine. This sympathetic surge increases energy
expenditure (EE), tissue catabolism, and
mobilization of protein, fat, and carbohydrates.
Immobility and delays in the administration of
nutritional support exacerbate these effects of
increased sympathetic outflow. Other untoward
effects include hyperglycemia, poor wound healing,
decreased serum proteins, increased carbon dioxide
(CO2) production, release of inflammatory
mediators, and depressed immune function.
After neurologic injury, the ensuing state of
cardiac hyperdynamism increases oxygen consumption
and caloric requirements. Studies using indirect
calorimetry demonstrate that patients with severe
head injury are hypermetabolic and hypercatabolic.
The resting energy expenditure (REE) can increase
40% to 165%. This increase has been demonstrated in
patients who are treated both with and without
steroids. In addition, delayed gastric emptying,
bacterial translocation, and altered vascular
permeability with resultant intestinal edema and
malabsorption also occur.
Patients with neurologic
injury. Patients who have head injury
exhibit changes consistent with hypermetabolism,
hypercatabolism, hyperglycemia, suppressed immunity,
and a generalized inflammatory response. Nutritional
support should satisfy these physiologic demands.
Patients who have suffered
a stroke. These patients also exhibit
hypermetabolic physiology. Although it has been
suggested that protein supplementation beyond normal
nutritional support after stroke may improve
outcome, recent trials have not supported this
strategy.
Patients who are obese.
Recent estimates indicate that approximately 40% of
adults in the United States are overweight. Patients
who are obese are more likely to manifest comorbid
medical conditions including hypertension, coronary
artery disease, diabetes mellitus, pulmonary
disease, and gout. Critical illness is likely to
result in protein malnutrition, inhibition of
lipolysis, and preferential use of carbohydrate
substrate for gluconeogenesis. It has been suggested
that patients who have actual body weight (ABW) in
excess of 125% of ideal body weight (IBW) may
benefit from diets that are hypocaloric and high
protein in structure. The goal for obese patients is
to provide adequate protein intake to meet but not
exceed metabolic demands. Ideally, the nutritional
supplementation will contain enough protein to keep
the patient in a positive nitrogen balance, but
sufficient relative calorie deficit to encourage a
net increase in lean body mass (LBM) and a loss of
total body fat.
II. Nutritional
assessment
Delivery of appropriate metabolic
support begins with an assessment of the patient's
nutritional status. It is important to identify
patients who present with subtle signs of
deficiencies in caloric, protein, vitamin, or trace
metal intake. These patients should be considered
candidates for earlier and more aggressive support.
Definitions. The
malnourished state exists when intake does not meet
nutritional demands. When approximately 10% of LBM
is lost, moderate protein energy malnutrition
exists. A loss of 20% of LBM indicates severe
protein malnutrition and describes a severely
underweight patient. When more than 35% of LBM is
lost, it is likely that irreversible changes leading
to death have occurred.
History. In most
cases, a history of recent unintentional weight loss
(5% LBM in 1 month or 10% LBM in 6 months) raises
the possibility of malnutrition. A careful social
history may demonstrate vitamin or mineral
deficiencies associated with alcoholism or other
substance abuse. Patients who have renal failure
lose amino acids, vitamins, and trace metals during
dialysis. Patients who have cancer may have
deficiencies owing either to the underlying disease
or to chemotherapy (e.g., methotrexate). Other risk
factors for malnutrition include age >75 years,
homelessness, nothing by mouth for more than 3 days,
major surgery, corticosteroid administration, renal
dialysis, malabsorption syndromes, and chronic
disease states, especially cancer, stroke, and
acquired immunodeficiency disease.
Physical examination.
Caloric intake can be assessed by the amount of fat
in the extremities, buttocks, and buccal fat pad.
The adequacy of protein intake can be evaluated from
the bulk of the extremity muscles, grip strength,
and size of the temporal muscle. Vitamin
deficiencies may manifest themselves as changes in
skin texture and hair quality and texture,
cheilosis, glossitis, or loss of vibration and
position sense. Examination of the head and neck may
reveal xerostomia, malocclusion, odynophagia,
dysphagia, esophagitis, or other findings suggestive
of difficulty with eating.
Anthropometrics.
Measurements are used to estimate the stores of body
fat and protein. Body fat is approximated by the
thickness of the triceps skin fold (TSF), and
protein status is estimated by the mid-arm muscle
circumference (MAMC).
MAMC = mid-arm circumference - fat
MAMC = mid-arm circumference - (0.314 x TSF)
These data are then compared with normal values to
determine the patient's nutritional status. Two
possibly incorrect assumptions are that body fat is
uniformly distributed and that population standards
apply to patients who are critically ill. In fact,
anthropometric measurements are generally invalid in
patients who are critically ill owing to anasarca.
Adjustments in the estimations can be based on IBW
calculated from the measurements of height and
weight.
Biochemical measurements.
Various biochemical and metabolic measurements have
been studied as potential indices of nutritional
status. Although many of these tests have
significant value in assessing either stable
patients who are scheduled for elective surgery or
those well on the way to recovery, their
applicability to patients who are critically ill has
not been demonstrated. Trends between the same
patient's measurements taken on different days can
be used if other factors are relatively stable.
Plasma proteins. Plasma
protein levels depend on hepatic synthetic function
and the availability of substrate. Unfortunately, a
decrease in level is not specific because the
biologic half-life, the catabolic rate, and a
variety of nonnutritional factors can alter plasma
protein levels. For example, expansion of the
extracellular fluid compartment results in a
reduction in albumin concentration. In addition,
some serum protein levels decrease promptly in
response to trauma, sepsis, or severe illness as a
result of fluid shifts, alterations in capillary
permeability, and changes in rates of synthesis and
degradation. Liver disease, nephrotic syndrome,
eclampsia, and protein-losing enteropathies are
additional causes of hypoproteinemia.
a. Albumin has a
half-life of approximately 18 days. It is the most
commonly used test to diagnose protein-calorie
undernutrition. Depending on measured albumin alone,
however, can lead to delays in treating nutritional
deficits because plasma levels can be maintained for
a long time owing to the long half-life. In critical
illness, albumin may also remain low until the
remission of the inflammatory response despite
adequate nutritional intake. Serum albumin levels
have been shown to be good predictors of sepsis and
major infection in surgical patients. However, serum
albumin has not been correlated with either
morbidity or mortality in the setting of severe
neurologic injury.
b. Transferrin has a
half-life of approximately 8 days. Therefore,
transferrin levels reflect acute changes in
nutritional status more accurately than albumin. Low
serum transferrin is indicative of protein
malnutrition. Iron deficiency, pregnancy, and
hypoxia stimulate synthesis of transferrin whereas
chronic infection, sepsis, and iron overload
decrease transferrin levels.
c. Thyroxine-binding
prealbumin and retinol-binding protein (RBP)
have a half-life of 2 to 3 days and 8 to 12 hours,
respectively. RBP complexes with prealbumin in the
circulation. The resultant compounds are much more
sensitive to short-term alterations in protein and
total caloric intake; low levels can return to
normal after only 3 days of adequate nutritional
support. While serum levels of the RBP-prealbumin
complex reflect acute changes in nutritional status
and indicate the adequacy of nutritional
supplementation, many nonnutritional factors can
affect these levels as well. Infection and trauma
depress prealbumin levels. Stress and vitamin A
deficiency decrease the concentration of RBP; renal
failure can cause erroneous elevation of RBP. The
short half-lives and the myriad of factors
influencing their serum concentrations have limited
the clinical significance and usefulness of these
proteins.
Immunologic functions.
Total lymphocyte count (TLC) and reactivity to skin
test antigens are immunologic functions that can
assess nutritional status, but they are not
routinely used in patients who are critically ill.
Any stressful situation, especially any disease
process that requires a stay in the ICU, can depress
cellular immunity, leading to a nonspecific test
result.
III. Estimation of
energy requirements
Because all of the previously
mentioned tests measure nutritional status
indirectly, other more sensitive and specific
techniques have been developed to assess nutritional
status.
Predictive equations
Harris-Benedict equation.
REE is the energy requirement at rest and can be
estimated using the Harris-Benedict equation as
follows:
Women: REE (kcal/day) = 65 + 9.6W + 1.8H - 4.7A
Men: REE (kcal/day) = 66 + 13.7W + 5H - 6.8A
where W = ABW in kilograms or IBW in kilograms if
the patient is edematous; H = height in centimeters;
and A = age in years. If the patient is obese, an
adjusted IBW should be used as follows:
Adjusted IBW = IBW + 0.25 (ABW - IBW)
Adjustments to REE. EE
can vary greatly from daily resting energy
requirement in active subjects. Hospitalized
patients typically require 30 kcal/kg/day in the
absence of severe illness or obesity. Fever
increases the REE by approximately 10% above
baseline for each degree Celsius rise in
temperature. Brain injury induces a hyperdynamic
state. Elevated EE is usually associated with total
body surface area burns of 20% to 40%. Figure 21-1
shows the EE as the percentage above normal for
several disease states. A common practice is to
adjust the estimated REE for the state of
hypermetabolism that characterizes patients who are
critically ill. Usual correction factors are as
follows:
Fever: REE × 1.1 (for each 1C above normal)
Mild stress: REE 7times; 1.2 - 1.3
Moderate stress: REE 7times; 1.4 - 1.5
Severe stress: REE 7times; 1.6 - 1.8
Using predictive equations in patients who are
critically ill has a potential limitation. The
Harris-Benedict equation was determined using
healthy volunteers as test subjects. The validity of
applying this equation to patients who are
critically ill has been questioned.
Indirect calorimetry.
Indirect calorimetry relies on the measurement of
oxygen consumption (Vco2) and the
production of carbon dioxide (Vco2). The
oxygen consumed in a given period of time is the
amount required for oxidation of carbohydrate, fat,
and protein.
Simultaneously, CO2 is produced from the
oxidation of carbohydrate, fat, and protein. If the
patient inspires a known concentration of O2
and CO2, measurements of Vco2
and Vco2 can be made. A metabolic
computer can then calculate the REE and the
respiratory quotient (RQ). Normal RQ is 0.8. An
elevated RQ indicates overfeeding or excess
carbohydrate in the diet.
Figure-1. Energy
expenditure as a percentage above normal in
several disease states |
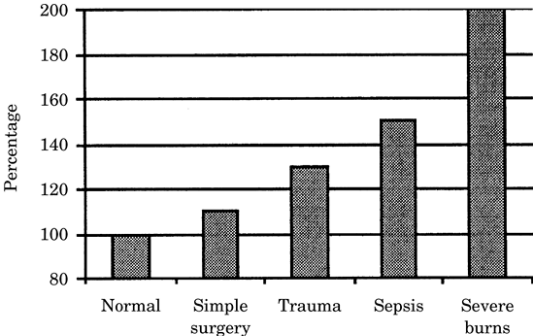 |
Usually, measurements taken over a minimum period of
20 to 30 minutes generate better baseline values.
Indirect calorimetry can be performed only on
patients who have fraction of inspired oxygen (Fio2)
requirements of 50% or less. To account for
sedation, ICU activity, dietary-induced
thermogenesis, anabolism, and the work of breathing,
an extra percentage is added to the measured EE.
Examples of usual correction factors are as follows:
Thermogenesis: 5% to 10%
ICU activity: 10%
Anabolism: 5% to 10%
Sedation: 0% to 30%
REE can be calculated using indirect calorimetry:
REE (kcal/day) = (3.9 x [Vo2] + 1.1 x [Vco2])
x
1.44
The Fick equation and mixed venous oxygen content
(Cvco2). The 24-hour EE for an ICU patient is
approximately 7 times the Vco2, calculated using
measured arterial oxygen content (Caco2), mixed
venous oxygen content (Cvco2), and the cardiac
output (CO). The arterial oxygen saturation (Sao2)
and mixed venous oxygen saturation (Svco2) should be
measured at the same time that CO is measured.
Cao2 - Cvo2 = Hb (g/dL) x1.39
x (Sao2 - Svo2)
Vo2 (mL/minute) = (Cao2 - Cvo2)x 10
x CO
(L/minute)
24-hour EE (kcal/day) = 7 x Vo2
These "spot" measurements should be performed
for several days in succession to establish an
accurate trend.
IV. Estimation of protein requirements
The daily protein requirements are usually estimated
first and measured later.
Predictive equations. The goal of protein
supplementation is to decrease the degree of loss of
LBM because there are no protein stores in the body.
Approximate protein requirements can be estimated on
the basis of the patient's weight, degree of
illness, and extent of organ system failure.
High-dose steroids, which are often given to
patients after neurologic insult, also increase
protein requirements because of catabolism.
Normal protein requirement: 0.8 to 1 g
protein/kg/day
Mild stress requirements: 1 to 1.2 g protein/kg/day
Moderate stress requirements: 1.2 to 1.4 g
protein/kg/day
Severe stress requirements: 1.4 to 1.6 g
protein/kg/day
Patients in renal failure on hemodialysis: 1.2 g
protein/kg/day
Patients who have encephalopathy: 0.8 g
protein/kg/day
Studies of patients with head injury receiving 1 to
1.5 g protein/kg/day have still reported negative
nitrogen balances. Current recommendations are to
provide 2 g protein/kg/day if renal function is
normal and to reassess the nitrogen balance in a few
days.
Postsurgical patients typically require 2 g
protein/kg/day.
Nitrogen balance. Nitrogen balance is used to assess
the adequacy of caloric and/or protein intake and
whether the anabolic state has been achieved as a
result of nutritional therapy.
Nitrogen balance equals nitrogen intake minus
nitrogen output. Total nitrogen output equals urine
urea nitrogen from a 24-hour urine collection and 2
to 4 g/day of nonurinary losses. In patients who are
critically ill, this may be as much as 6 g/day owing
to blood loss, increased mucosal sloughing, and
increased fecal nitrogen loss from diarrhea. Because
protein is 16% nitrogen, each gram of urinary
nitrogen represents 6.25 g of degraded protein.
Nitrogen balance = (protein intake/6.25) - (urine
urea nitrogen + [2 or 4])
Measurements of nitrogen balance may not be reliable
in patients who have renal and hepatic failure owing
to altered protein synthesis and clearance. For
patients who are in renal failure, the creatinine
(Cr) clearance must be >50 mL/minute to achieve a
valid result.
V. Macronutrients
Carbohydrates. As long as the diet provides adequate
energy and protein, there is no specific requirement
for dietary carbohydrate, but current
recommendations are to provide 50% to 60% of total
calories as carbohydrates. Much of this is used by
the central nervous system, which relies on glucose
as its primary fuel source.
Fat. The goals of lipid supplementation include
providing for essential fatty acids, promoting
nitrogen sparing, and offering a balanced approach
to fulfilling energy requirements. Increased fat
decreases the incidence of hepatic steatosis and the
production of CO2 from a high-carbohydrate diet.
Lipid oxidation as an energy source is increased in
the patient who is critically ill. The
recommendation is therefore to provide 30% to 40% of
the total calories as lipid. A minimum of 3% of the
kilocalories delivered as fat should be linoleic
acid to prevent a deficiency of essential fatty
acids.
Protein. The goal of protein supplementation is to
provide substrate for cellular protein synthesis and
the maintenance of LBM. Protein requirements
increase if excessive losses from the GI tract,
skin, or draining wounds occur. It is often
difficult to achieve positive balance in patients
who are critically ill, but the effort to minimize
negative nitrogen balance should be made.
Fluid. Nonhospitalized patients consume
approximately 1 mL of free water/kcal ingested. With
a normal diet, this is approximately 30 to 50 mL/kg
of body weight per day. Patients who are
hospitalized typically require 30 to 35 mL/kg/day.
The patients' underlying medical condition and
comorbidities must be considered when replacing
fluids. Patients should be adequately volume
resuscitated before initiating parenteral nutrition.
When calculating free water replacement in patients
receiving enteral nutrition, it is important to
remember to subtract the free water in the enteral
formula from the total daily volume of free water.
VI. Micronutrients
Because the response to injury is characterized by
greatly increased energy demands and clinical
malnutrition, appropriate nutritional support might
prevent the multisystem organ failure that often
occurs in patients who are critically ill. The gut
is now being seen as an important metabolic organ
that has a critical need for certain substrates.
Clinical trials are being conducted to evaluate
diets enriched with immunomodulatory components.
Although the use of these components has been
advocated, currently their benefits remain
controversial.
Vitamins. Vitamins are involved in metabolism, wound
healing, and immune function. They are essential and
cannot be synthesized by the body. The 12 essential
vitamins that should be supplied daily are divided
into fat- and water-soluble categories. Recommended
dietary allowances of vitamins are listed in Table-1.
Trace nutrients. Deficiencies in the nine essential
trace elements can lead to problems in several organ
systems including insulin resistance, myopathy,
increased susceptibility to infections, and
pancytopenia. Recommended dietary allowances of
trace elements are listed in Table-2.
Table-1. Recommended dietary allowance of
vitamins for a healthy 25- to 50-year-old man |
Vitamin |
Amount |
Vitamin |
Amount |
Vitamin A |
1,000 mcg |
Vitamin D |
5 mcg |
Vitamin E |
10 mg |
Vitamin K |
80 mcg |
Vitamin C |
60 mg |
Thiamine |
1.5 mg |
Riboflavin |
1.7 mg |
Niacin |
19 mg |
Vitamin B6 |
2 mg |
Folate |
200 mcg |
Vitamin B12 |
2 mcg |
|
|
For patients in renal failure, zinc and chromium
should be either reduced or omitted because they are
excreted in the urine. Similarly, in the presence of
biliary tract obstruction, the intake of copper and
manganese is either reduced or omitted because both
are excreted primarily in the bile.
Decreased serum zinc levels and increased urinary
zinc losses have been reported after a head injury,
although the mechanism and clinical significance are
unknown. No other specific changes in vitamin and
mineral metabolism have been identified after
neurologic injury.
Glutamine. The primary fuel for the intestine,
glutamine is important in maintaining intestinal
structure and function, preventing mucosal atrophy
during starvation, decreasing bacterial
translocation, and stimulating the intestinal immune
system in sepsis and stress. Glutamine may also
decrease intestinal injury during ischemia by
preserving the level of glutathione in the gut.
Table-2. Recommended dietary allowance of trace
elements for a healthy 25- to 50-year-old man |
Element |
Oral
|
Intravenous
|
Zinc |
10-15 mg |
2.5-4.0 mg |
Chromium |
50-290 mcg |
10-15 mcg |
Copper |
1.2-3 mg |
0.5-1.5 mg |
Manganese |
0.7-5 mg |
0.15-0.8 mg |
Selenium |
50-200 mcg |
40-120 mcg |
Molybdenum |
|
200 mcg |
Arginine. Arginine improves wound healing,
stimulates immune function by increasing the
production of natural killer and helper T cells, and
enhances intestinal absorption and proliferation.
Arginine is also responsible for the synthesis of
nitric oxide, which maintains the integrity of
microvascular structure in the gut after injury.
Omega-3 fatty acids. The omega-6 fatty acids have
been shown to impair the immune response by
generating degradation products that suppress T cell
and macrophage activity. Replacement of dietary
omega-6 fatty acids with omega-3 fatty acids might
down regulate several aspects of the inflammatory
response and thereby improve immune function. A few
reports have indicated, however, that omega-3 fatty
acids may interfere with wound healing. Balance
should therefore be maintained between the two fatty
acids.
Dietary nucleotides. Dietary nucleotides are
important for intestinal structure and function. In
animals, the addition of dietary nucleotides to
total parenteral nutrition (TPN) diminishes the
intestinal mucosal atrophy. Ribonucleic acid
nucleotides have also been shown to stimulate the
immune system by promoting the development of T
lymphocytes.
Growth hormone. In patients who are critically ill,
growth hormone has beneficial anabolic effects. It
is associated with the mobilization of fat stores as
an energy source and the enhancement of whole-body
protein stores in trauma patients. TLC and serum
albumin and transferrin levels have also increased
after the administration of growth hormone.
Intestinal function is also enhanced directly by the
binding of growth hormone to growth hormone
receptors in the gut and indirectly by the growth
hormone's stimulation of insulin-like growth factor.
Anabolic steroids. Anabolic steroids are sometimes
used as a replacement for growth hormone because of
their beneficial effect on the deposition of lean
tissue. They cause less hyperglycemia than growth
hormone and also cost substantially less.
VII. Timing and route of feeding
Although some patients can safely tolerate protein
and calorie deprivation for several days,
nutritional support should not be delayed in
patients who are critically ill and are not expected
to have any oral intake for a prolonged period.
Nutritional support should also be administered
promptly to patients who will have huge caloric
requirements from burns, sepsis, trauma, or head
injury. The decision as to which route (enteral or
parenteral) to use involves assessing the functional
capacity of the gut. It is important to remember
that enteral feeding and parenteral feeding are not
mutually exclusive; combinations are often
appropriate. Each has specific advantages and
complications, but overall, it appears that early
nutritional support is more important than the route
chosen.
Enteral feeding. If the GI tract is functional,
enteral feeding is preferred because it is easier,
safer, and less expensive. Auscultation of the
abdomen for bowel sounds as an indication of bowel
function can be misleading. The absence of bowel
sounds does not mean the absence of bowel function.
If no air is present, there will be no bowel sounds,
but the small bowel will likely still have
peristalsis and absorptive function.
Contraindications for the use of tube feedings
include ileus, intestinal obstruction, and the need
for total bowel rest. Studies in animals have shown
that villus atrophy increases intestinal
permeability, and bacterial translocation occurs
after parental nutrition and gut disuse. Although
this has yet to be demonstrated in humans, the
occurrence of the sequence has been extrapolated
from clinical cases in which bacteria from the gut
have been cultured in patients who are critically
ill and had no clear focus of abdominal infection.
Types of formulas. The selection of an enteral
formula is based on the patient's digestive capacity
and specific nutritional needs. Table-3 lists
some enteral formulas and their nutritional
components.
Polymeric contains intact nutrients and requires
normal digestion and absorption.
Fiber enriched is frequently used in patients who
have diarrhea or constipation.
Calorically dense is used in patients who have
cardiac, renal, or hepatic failure and when fluid
restriction is required.
Elemental contains one or more partially digested
macronutrients and is used in patients who have
compromised GI function.
Modular is composed of individual nutrient modules
to produce a formula customized to meet a patient's
specific needs. These formulations have increased
caloric and protein concentrations. They can also be
used to modify a preexisting commercial formula to
add to the caloric and/or protein density.
There has been a great deal of interest in the
immunomodulating potential of nutrients such as
omega-3 fatty acids, branched-chain amino acids,
nucleotides, arginine, and glutamine. While these
nutrients do change the intermediate metabolic
pathways that affect cellular survival invitro, thus
far randomized trials have not demonstrated any
difference in length of hospital stay, morbidity, or
mortality.
Table-3. Enteral formulas and their components
|
|
|
|
|
Composition per
liter (g) |
Product |
Formula type |
kcal/mL |
mosmol |
Protein |
Fat |
CHO |
Osmolite |
Polymeric |
1.06 |
300 |
37 |
38 |
145 |
Osmolite HN
Plus |
|
1.2 |
360 |
56
|
39 |
158 |
Jevity |
Fiber-enriched |
1.06 |
300 |
44 |
36 |
151 |
Jevity
Plus |
|
1.2
|
450
|
56
|
39
|
175 |
Glucerna
|
|
1
|
375
|
42
|
56
|
94 |
Two Cal HN |
Calorically dense |
2
|
690
|
84
|
91
|
217 |
Peptamen |
Peptide-based (semielemental) |
1
|
270
|
40
|
39
|
127 |
Peptamen VHP |
|
1
|
300
|
62
|
39
|
104 |
Crucial |
|
1.5
|
490
|
94
|
68
|
135 |
Vivonex
Plus |
Elemental |
1
|
650
|
45
|
6.7
|
189 |
CHO, carbohydrate. |
Routes of enteral feeding
Gastric. Gastric
feeding can be established either by the insertion
of a feeding tube through the nose to the stomach or
by the surgical insertion ("open"
or laparoscopically) of a gastrostomy tube. This
method takes advantage of the reservoir capacity of
the stomach, but many patients, especially those who
have elevated intracranial pressure, might not
tolerate this owing to delayed gastric emptying,
impaired gag reflex, and/or gastric atony.
Duodenal. The proposed advantage is that there is
reduced risk of reflux and aspiration, but,
unfortunately, there is no evidence to support this
hypothesis. Patients can still aspirate, even with
jejunal feeds. Feedings given into the duodenum must
be as continuous infusions because large boluses are
not tolerated.
Percutaneous enteral tubes. Gastric (percutaneous
endoscopic gastrostomy [PEG]) and jejunal
(percutaneous endoscopic jejunostomy [PEJ]) tubes
can be inserted percutaneously under either
fluoroscopic or endoscopic guidance. PEG tubes allow
enteral feeding of patients who have dysphagia or
altered mental status. PEJ tubes allow enteral
nutrition with a reduction in gastric residuals.
Neither PEG nor PEJ feeding prevents aspiration.
Initiation and advancement. Before initiating
enteral feeding, proper placement of the enteral
tube should be ascertained radiographically. An
abdominal radiograph is performed to locate the distalmost portion of the enteral tube.
Isotonic solutions can be started slowly at full
strength and then the rate advanced as tolerated
until the goal rate is achieved. Hypertonic
solutions should be diluted to isotonicity and the
concentration advanced to full strength before the
infusion rate is increased. These strategies help
guard against diarrhea and electrolyte disturbances.
Enteral feeding through the stomach (nasogastric or
PEG) is typically advanced while monitoring gastric
residuals. Patients requiring intensive care often
have delayed gastric emptying and gastric
distention, which predispose them to aspiration.
Enteral feeding may begin at 10 to 15 mL/hour; and
the rate is increased by 10 to 15 mL/hour every 8
hours until a predetermined goal rate is reached. If
the feeds are delivered to the stomach, gastric
residuals should be checked every 6 hours and
infusions held for residuals of >200 mL.
Emesis, gross abdominal distention with absent bowel
sounds, and radiographic evidence of bowel
obstruction necessitate alterations in feeding.
Complications of enteral feeding
Mechanical. Misplaced nasal feeding tubes might end
up in the bronchus or in the brain in patients who
have fractures of the cribriform plate. There is
also a higher incidence of epistaxis and sinusitis
because the tube passes through the nares.
GI. Nausea and vomiting might occur from postinjury
ileus and gastric atony. Diarrhea can be a problem
because of the concentration, osmolarity, or volume
of the administration of the tube feeds; the
presence of lactose, fat, or gluten; low residue; or
bacterial contamination. Diarrhea may also be caused
by either the lack of fiber or an intestinal
obstruction and may lead to either free water
deficits or dehydration.
Pulmonary. Pulmonary aspiration is probably the most
common serious, potentially fatal complication of
enteral nutrition. The reported incidence is highly
variable (0% to 95%), depending on the population of
patients studied and the definition of aspiration
because chemical evidence of aspiration is more
common than clinically diagnosed aspiration
pneumonia. The presence of tube feeds in the
pulmonary secretions can be detected by checking for
glucose or adding food coloring to the feeds and
checking the secretions for a color change.
Metabolic. Osmolarity and volume status must be
carefully monitored to avoid hyperosmolarity and
volume overload. Electrolyte imbalance (e.g.,
hyponatremia) is common, especially in patients who
have a head injury and are at risk for the syndrome
of inappropriate antidiuretic hormone secretion
(SIADH) or diabetes insipidus. Hypophosphatemia,
hypokalemia, and hypomagnesemia occur commonly when
feeding is begun again.
Parenteral feeding. TPN should be initiated when gut
failure is demonstrated. Before initiating
parenteral nutrition, the patient's total energy
requirements, total protein requirements, daily
fluid requirements, and the proportion of calories
to be delivered as fat must be determined.
Parenteral solutions. There are three elemental
nutrients in parenteral nutrition.
1. Dextrose is available in concentrations ranging from
10% to 70%. The higher the percentage of dextrose,
the higher the osmolarity and the greater the need
for central access. Only solutions with <10%
dextrose are considered safe for peripheral venous
administration because solutions with an osmolarity
of >900 mOsm/L can cause either sclerosis or
phlebitis.
2. Amino acids provide for protein requirement.
3. Fat emulsions are rich in linoleic acid. The
infusions are given over several hours, and the rate
of infusion should be adjusted for patients
receiving propofol for sedation. Propofol is in a
fat emulsion and provides the equivalent of 1.1
kcal/mL of fat calories.
4. Additives such as electrolytes, vitamins, and trace
elements are added directly to the dextrose and
amino acid infusion.
Routes of parenteral feeding. Intravenous calories
can be given peripherally or centrally.
1. Peripheral access for administration of TPN should
be used only for short-term nutritional support. The
hyperosmolarity and low pH of TPN predispose small
veins to thrombophlebitis. It is also difficult to
provide the necessary caloric requirements by way of
this route owing to the limitations imposed by
dextrose (maximum 10%) and amino acid
concentrations. Long peripheral lines that extend
into a central vein can be used as a central line
and do not have the same limitations from the
dextrose and amino acid concentrations.
2. Central access should be used when longer periods of
parenteral feeding are necessary or if the patient
is seriously ill.
Initiation and advancement. Parenteral nutrition is
usually started at a slow rate of infusion and then
the rate is advanced every 12 to 24 hours until the
goal rate is achieved. When the infusion is
discontinued, the rate should be tapered so that
hypoglycemia does not result from the increase in
insulin production stimulated by the parenteral
nutrition.
Complications
Mechanical. Significant venous thrombosis, which can
usually be diagnosed by ultrasound or the injection
of radiopaque contrast, requires the removal of the
catheter and heparinization. Catheter infections
must always be suspected. Meticulous catheter care,
a high index of suspicion, and a low threshold for
removal are essential.
GI. Elevated transaminases are common at the
beginning of the administration of TPN, but they
should not persist for long. Fatty liver might
develop from the excess carbohydrates or total
calories in the diet. Cholelithiasis or gall bladder
sludge might be secondary to changes in bile
composition as well as the decreased frequency of
gallbladder contractions. These usually resolve
after enteral alimentation has been initiated.
Metabolic. Elevations in glucose can occur early
from relative insulin resistance and might require
an infusion of insulin. Hypercapnia can result from refeeding a malnourished patient who cannot
adequately increase minute ventilation. Decreasing
the daily calories or replacing dextrose with fat
can reduce carbon dioxide production. Any
electrolyte abnormality can occur, so that daily
adjustments must be made in conjunction with the
laboratory results. Suggested laboratory monitoring
guidelines are listed in Table 21-4. Vitamin K is
the most common vitamin deficiency, because it is
not contained in multivitamin preparations.
Monitoring. While receiving enteral or parenteral
nutrition, the patient must be monitored for changes
in body composition, blood chemistry, blood glucose,
triglycerides, and protein synthesis.
Table-4. Suggested monitoring guidelines for
enteral and parental nutrition |
Schedule |
Enteral |
Parenteral |
Baseline |
Electrolytes, BUN,
Cr, Ca, Mg, PO4, glucose, albumin |
Electrolytes, BUN, Cr, Ca, Mg, PO4,
glucose, liver function tests, triglycerides,
cholesterol, albumin |
Daily |
Intake and output,
weight |
Intake and output,
weight |
Daily until stable;
then 2 to 3 times/week |
Electrolytes, BUN,
Cr, glucose |
Electrolytes, BUN,
Cr, glucose |
Every other day
until stable; then 1 to 2 times/week |
Ca, Mg, PO4 |
Ca, Mg, PO4 |
Every 10-14 days |
Albumin |
Liver function tests,
albumin, triglycerides |
Weekly |
PT, prealbumin |
PT, prealbumin |
BUN, blood urea nitrogen; PT, prothrombin time; Cr,
creatinine; PO4, phosphate. |
Daily weight and the total volume of the patient's
intake and output need to be monitored in addition
to assessing other markers of hydration and volume
status.
Electrolytes (Na, K, Cl, Mg, Phosphate, and Ca) and
markers of renal function (blood urea nitrogen and
creatinine) should be monitored routinely.
Intensive glycemic therapy in patients who are
critically ill has been shown to reduce morbidity
and mortality to a significant extent. Maintaining
blood glucose levels of 80 to 110 mg/dL in all
patients who are critically ill, regardless of
preexisting diabetes mellitus, resulted in a
relative morbidity and mortality risk reduction of
approximately 40%. Recent studies also suggest that
tight glycemic control in patients who have isolated
severe brain injury may reduce secondary central and
peripheral neurologic injury.
Hypertriglyceridemia is a potential complication of
sedation with continuous infusion of propofol
because of the lipid carrier solution. Escalating or
preexisting elevated triglyceride levels may
predispose patients to pancreatitis and therefore
necessitate alternate sedation strategies.
Refeeding syndrome. Patients who are severely
malnourished could have a physiologic response to
carbohydrate utilization. During starvation, insulin
production decreases in the absence of
carbohydrates. Protein and fat are preferentially
used to meet metabolic demands. As a result,
intracellular electrolytes are depleted, especially
phosphate. When carbohydrates are administered,
insulin causes electrolyte and fluid shifts. This
results in hypokalemia, hypomagnesemia,
hypophosphatemia, and fluid retention. Without
phosphate, adenosine triphosphate cannot be
generated. In the most severe cases, arrhythmias,
heart failure, respiratory failure, seizures, or
death may occur. Prolonged fasting, prolonged
intravenous hydration, chronic malnutrition, chronic
alcoholism, and anorexia nervosa are a few of the
risk factors for the refeeding syndrome. Closely
monitoring electrolytes and vital signs can prevent
a potentially fatal complication of refeeding
patients who are severely malnourished.
|