| Head Injury |
Supratentorial Tumors |
Posterior Fossa Surgery|
Intracranial Aneurysms
|Ischemic Cerebrovascular Diseases
|Neuroendocrine Tumors
|Epilepsy-Awake
Craniotomy-Intraoperative MRI
|Spinal Cord Injury and Procedures
|Pediatric Neuroanesthesia
|Neurosurgery in the Pregnant
Patient
|Management of Therapeutic
Interventional Neuroradiology
|Management in Diagnostic
Neuroradiology
|

Anesthetic
Management of Head Trauma
Head trauma or traumatic brain injury (TBI) is one
of the most serious, life-threatening conditions in
trauma victims. Prompt and appropriate therapy is
necessary to obtain a favorable outcome.
Anesthesiologists manage these patients throughout
their perioperative course, taking them from the
emergency room to the neuroradiology suite, the
operating room, and the neurointensive care unit.
The perioperative management of patients with head
injuries focuses aggressively on the stabilization
of the patient and the avoidance of the systemic and
intracranial insults that cause secondary neuronal
injury. These secondary insults, while potentially
preventable and treatable, can complicate the course
of patients with head injuries and adversely affect
outcome.
I. Head injury
practice guidelines
Evidence-based guidelines for the
management of severe TBI were published in 2000
after an extensive review of the literature. Three
standards on the basis of Class I evidence and
several guidelines on the basis of Class II evidence
were recommended. In March 2006, the Brain Trauma
Foundation and the Congress of Neurological Surgeons
published new guidelines. This review presents
literature-based recommendations for the surgical
management of TBI.
II. Classification of
head injury
Head injury is classified into
primary injury and secondary injury. This
classification is useful when considering
therapeutic strategies.
Primary injury
is the damage produced by a direct mechanical impact
and the acceleration-deceleration stress onto the
skull and brain tissue, resulting in skull fractures
(cranial vault, skull base) and intracranial
lesions. The intracranial lesions are further
classified into two types: diffuse injury and focal
injury.
Diffuse brain injury
includes two categories.
Brain concussion is
loss of consciousness lasting <6 hours.
Diffuse axonal injury
is traumatic coma lasting >6 hours.
Focal brain injury
includes the following types:
Brain contusion is
usually located either below or opposite the region
of impact.
Epidural hematoma is
often caused by skull fracture and laceration of the
middle meningeal artery.
Subdural hematoma is
usually caused by the tearing of the bridging veins
between the cerebral cortex and draining sinuses.
Acute subdural hematoma is often associated with
high mortality.
Intracerebral hematoma
is usually located in the frontal and temporal lobes
and visualized as a hyperdense mass on a computed
tomographic (CT) scan. Brain tissue destroyed by the
primary impact will not be saved. Therefore,
functional outcome is improved by prompt surgical
intervention and medical therapy.
Indications for surgery.
Presently, no definitive therapeutic measure exists
to treat diffuse axonal injury. Most open and
depressed skull fractures and compound skull
fractures with dural laceration require early
surgical repair. Uncomplicated basal fractures
usually do not require operation. The presence of
compression of the basal cisterns from a brain
contusion indicates operative intervention because
of the risk of herniation (usually of the temporal
lobe). Intracranial hematoma is the most common
sequela of head trauma requiring surgical treatment.
Secondary injuries
develop within minutes, hours, or days of the
initial injury and cause further damage to nervous
tissue. The common denominators of secondary injury
are cerebral hypoxia and ischemia. Secondary
injuries are caused by the following disorders:
Respiratory dysfunction (hypoxemia, hypercapnia).
Cardiovascular instability (hypotension, low cardiac
output).
Elevation of intracranial pressure (ICP).
Biochemical derangements.
III. Pathophysiology
of head trauma
Comprehensive management requires
an understanding of the pathophysiologic responses
to TBI.
Systemic effects of head
trauma
Cardiovascular responses to
head trauma are commonly observed in the
early stage. They include hypertension, tachycardia,
and increased cardiac output. Patients with severe
head injuries and those suffering from multiple
systemic injuries with substantial blood loss,
however, may develop hypotension and a decrease in
cardiac output. Systemic hypotension (systolic blood
pressure of <90 mm Hg) at the time of admission to
the hospital is associated with significantly
increased morbidity and mortality.
Respiratory responses to head
trauma include apnea and abnormal respiratory
patterns. Respiratory insufficiency and spontaneous
hyperventilation often occur. Patients may also
suffer from aspiration of vomitus and central
neurogenic pulmonary edema.
Temperature regulation
may be disturbed, and hyperthermia, if it occurs,
can provoke further brain damage.
Changes in cerebral
circulation and metabolism.
In focal brain injury,
cerebral blood flow (CBF) and cerebral metabolic
rate for oxygen consumption (CMRo2)
decrease in the core area of injury and in the
penumbra, an area of hypoperfused tissue that
surrounds the damaged tissue. When ICP increases,
diffuse and more marked hypoperfusion and
hypometabolism ensue.
In diffuse brain injury,
hyperemia may occur. In most cases, however, CBF
decreases within a few hours of head trauma. The
combination of hypotension and impaired
autoregulation exacerbates cerebral ischemia. The
chemical-metabolic regulation of CBF may also be
impaired. The combination of these pathophysiologic
responses to head injury creates a complicated
management scenario.
Acute brain swelling and cerebral edema
Acute brain swelling is
provoked by a decrease in vasomotor tone and a
marked increase in the volume of the cerebral
vascular bed. In this situation, increases in blood
pressure can easily lead to further brain swelling
and an increase in ICP.
Cerebral edema
following head trauma is often a mixture of
vasogenic and cytotoxic types caused by blood-brain
barrier disruption and ischemia, respectively.
Following head trauma, both acute brain swelling and
edema ensue concurrently. When these pathologic
conditions occur in association with an intracranial
hematoma, the resultant intracranial hypertension
causes a further reduction in CBF with cerebral
ischemia. Eventually, intracranial hypertension, if
untreated, leads to herniation of the brain stem
through the foramen magnum.
Excitotoxicity. Head
trauma causes excessive release of glutamate from
neurons and glia, increasing the concentration of
glutamate in the cerebrospinal fluid (CSF). The
biochemical changes associated with the excessive
release of glutamate and the activation of glutamate
receptors are closely related to an increase in
intracellular calcium ion, which triggers a number
of events that lead to the damage. These include an
activation of phospholipase, protein kinase,
proteases, nitric oxide synthase, and other enzymes.
Activation of these enzymes also produces lipid
peroxidation, proteolysis, free radical formation,
deoxyribonucleic acid (DNA) damage, and finally,
neuronal death (Figure -1).
Figure-1. Excitotoxicity
in head injury. AMPA,
alpha-amino-3-hydroxy-5-methyl-4-isoxazoleproprionate;
NMDA, N-methyl-d-aspartate; [Ca]i,
intracellular Ca. |
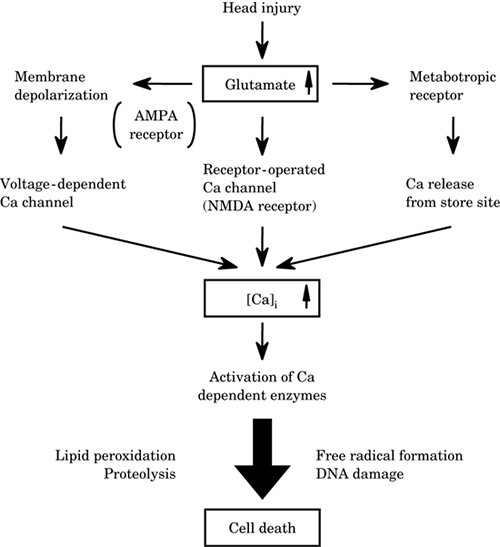 |
Inflammatory cytokines and mediators. The cytokines
are major mediators in the initiation of
inflammatory and metabolic responses to injury.
Cytokines increase in response to cerebral ischemia.
Interleukin-6 (IL-6) and tumor necrosis factor alpha
are known to be released after TBI. Patients who
have Glasgow Coma Scale (GCS) scores of <8 show a
higher and more sustained elevation of IL-6. The
cytokines released after TBI stimulate the
production of free radicals and arachidonic acids
and upregulate the activity of the adhesion
molecules, which produce disturbances in the
microcirculation. All of these changes contribute to
secondary brain injury.
IV. Emergency management
Initial assessment of the patient's condition
Neurologic assessment. There is usually little time
initially to evaluate the patient's neurologic
condition thoroughly. However, a quick neurologic
assessment can be performed while stabilizing the
patient to achieve adequate ventilation (oxygenation
and carbon dioxide [CO2] elimination) and
hemodynamic stability.
GCS (Table -1) is a simple and universally accepted
method for assessing consciousness and neurologic
status in patients with head injuries. The GCS has
low interobserver variability and is a good
predictor of outcome.
(1) GCS score of <8 characterizes severe head
injury.
(2) GCS score of 9 to 12 represents moderate injury.
(3) GCS score of 13 to 15 represents mild injury.
Pupillary responses (size, light reflex) and
symmetry of motor function in the extremities should
be quickly assessed.
Assessment of injuries to other organs. Trauma
patients often suffer from injuries to multiple
organ systems. Particular attention should be paid
to determine whether there is evidence of intrathoracic or intraperitoneal (intrapelvic)
hemorrhage. If bleeding is suspected, the thorax or
abdomen should be explored without delay.
Treatment of hemorrhagic shock takes precedence over
neurosurgical procedures. The patient's hemoglobin
must be measured immediately, and blood and
fresh-frozen plasma should be set up and made
available for infusion.
Establishment of airway and ventilation
Tracheal intubation.
The first step in emergency therapy is to secure an
open airway and ensure adequate ventilation. Because
all trauma patients are considered to have a full stomach and
frequently (approximately 10%) have an associated
cervical spine injury as well, cricoid pressure and
in-line stabilization of the cervical spine are used
during laryngoscopy and intubation.
If facial fractures and soft tissue edema prevent
direct visualization of the larynx, either
fiberoptic intubation or intubation with an
illuminated stylet may be attempted. In the presence
of either severe facial injuries or laryngeal trauma
a cricothyrotomy may be required. Nasal intubations
are avoided in the presence of a suspected basal
skull fracture, severe facial fractures, and
bleeding diathesis.
Table -1. Glasgow Coma Scale
|
Adult Scale |
Pediatric Scale
|
Parameter |
Score |
Parameter |
Score |
|
Eye opening |
Eye opening |
Spontaneously |
4 |
Spontaneously |
4 |
To speech |
3 |
To speech |
3 |
To pain |
2 |
To pain |
2 |
None |
1 |
None |
1 |
Best verbal response |
Best verbal responsea |
Oriented |
5 |
Oriented to place |
5 |
>5 y |
Confused |
4 |
Words |
4 |
>12 mo |
Inappropriate |
3 |
Vocal sounds |
3 |
>6 mo |
Incomprehensible |
2 |
Cries |
2 |
<6 mo |
None |
1 |
None |
1 |
|
Best motor response |
Best motor response in upper limbsa |
Obeys commands |
6 |
Obeys commands |
6 |
>2 y |
Localizes to pain |
5 |
Localizes to pain |
5 |
6 mo-2 y |
Withdraws from pain |
4 |
Normal flexion to
pain |
4 |
>6 mo |
Flexes to pain |
3 |
Spastic flexion to
pain |
3 |
<6 mo |
Extends to pain |
2 |
Extension to pain |
2 |
|
None |
1 |
None |
1 |
aScore highest appropriate for age.
|
Nasal intubation also adds risk to patients who have
basilar skull fractures because of the introduction
of contaminated material into the brain. Basilar
skull fractures are strongly suspected when
hemorrhage of the tympanic cavity, otorrhea,
petechiae on the mastoid process (Battle's sign),
and petechiae around the eyes (panda sign) are
observed.
Mechanical ventilation. As soon as the trachea has
been intubated, a nondepolarizing muscle relaxant is
administered and mechanical ventilation to a partial
arterial pressure of carbon dioxide (Paco2) of
approximately 35 mm Hg is instituted. Aggressive
hyperventilation (Paco2 of <30 mm Hg) should be
avoided unless transtentorial herniation is
suspected. Hypoxemia, if present, should be
corrected immediately. Hyperoxia may be recommended.
If massive aspiration is suspected, bronchial
suctioning using a fiberscope is advisable before
transferring the patient to either the
neuroradiology suite or the operating room.
Cardiovascular stabilization. Systemic hypotension
is one of the major contributors to poor outcome
after head trauma. When necessary, fluid
resuscitation is initiated immediately and inotropic
and vasopressor drugs are administered as required
to stabilize the blood pressure.
Fluid resuscitation. Hypovolemia is often masked by
a relatively stable blood pressure secondary to
either sympathetic hyperactivity or the reflex
response to increased ICP. Therefore, fluid
resuscitation should be guided not only by blood
pressure but also by urinary output and central
venous pressure (CVP).
Crystalloid and colloid solutions. Isotonic and
hypertonic crystalloid solutions and colloid
solutions may be given to maintain adequate
intravascular volume.
(1) Lactated Ringer's solution is slightly hypotonic
relative to plasma, which precludes the use of a
substantial amount. If administered, serum
osmolarity should be measured periodically. When
large-volume resuscitation with crystalloid is
required, an isotonic crystalloid, such as normal
saline, is preferable.
(2) Hypertonic saline (3%, 7.5%) can be beneficial
in small amounts after TBI. Large volumes may
produce a lethal increase in serum sodium
concentration.
(3) Hydroxyethyl starch (HES) and human plasma
products can be administered to maintain
intravascular volume for longer periods. No more
than 1.5 L of HES should be administered in
conjunction with careful monitoring of blood
coagulation. The incidence of coagulopathy in
patients with head injuries is approximately 20%,
and HES in large amounts is known to interfere with
blood coagulation. The reported incidence of
coagulopathy is less with pentastarch.
Blood and blood products. Patients who have a low
hematocrit may require a transfusion to optimize
oxygen delivery; the hematocrit ideally is
maintained above 30%. Children require special
attention because they can easily become hypovolemic
by losing large volumes of blood into an
intracranial or subgaleal hematoma or through a
scalp laceration, even without blood loss in other
organ systems.
Adverse effect of glucose-containing solutions.
Glucose-containing solutions are avoided because
hyperglycemia is associated with worsened neurologic
outcomes. Glucose should be used only to treat
hypoglycemia. The plasma level of 80 to 150 mg/dL is
desirable; values above 200 mg/dL should be avoided
and treated with insulin.
Inotropics and vasopressors. If the blood pressure
and cardiac output cannot be restored through fluid
resuscitation, the administration of intravenous
inotropic and vasopressor drugs may be necessary. An
infusion of either phenylephrine or dopamine is
recommended to maintain cerebral perfusion pressure
(CPP), the difference between the mean arterial
pressure (MAP) and the ICP, above 60 mm Hg.
Management of elevated ICP. The reduction of
elevated ICP and the maintenance of blood pressure
are crucial in the management of intracranial
hypertension because CPP is directly related to both
MAP and ICP.
Hyperventilation. When evidence of transtentorial
herniation in patients with severe head injuries
exists, hyperventilation to a Paco2 of 30 mm Hg
should be instituted because hyperventilation can
rapidly and effectively reduce ICP. Hyperventilation
was previously thought to be more effective in
children than in adults because of the idea that
pediatric patients, unlike adults, responded to TBI
with acute brain swelling from hyperemia. The
accumulation of
recent data has revealed, however, that hyperemia
may not occur as commonly in severe pediatric TBI.
The initial response of both adult and pediatric
patients to TBI is more often hypoperfusion.
Aggressive hyperventilation to a Paco2 of <30 mm Hg
can therefore aggravate ischemia through excessive
vasoconstriction. To avoid this risk, other
measures, including diuretic therapy, barbiturate
therapy, and CSF drainage, should be instituted. The
Paco2 is allowed to return toward normal as soon as
possible.
Diuretic therapy. Mannitol, 1 g/kg intravenously
(i.v.) infused over 10 minutes, is administered to
patients in whom transtentorial herniation is
suspected. In less acute cases, an infusion of 0.25
to 1 g/kg may be administered over 10 to 20 minutes
and repeated every 3 to 6 hours. The serum
osmolarity is monitored frequently and should not
exceed 320 mOsm/L.
Posture. A head-up tilt of 10° to 30° facilitates
cerebral venous and CSF drainage and lowers ICP.
This ICP-reducing effect is negated when systemic
blood pressure decreases.
Corticosteroids. Corticosteroids were previously
thought to be of value in reducing brain edema and
hence ICP in patients with head trauma. However,
recent reports have demonstrated worsened outcomes
with the use of corticosteroids. Steroids also
increase blood glucose levels, which can adversely
affect the injured brain. Corticosteroids,
therefore, have no place in the treatment of head
injury despite their proven efficacy in spinal cord
injury.
Barbiturates. Barbiturates are known to exert
cerebral protective and ICP-lowering effects.
High-dose barbiturate therapy may be considered in
patients with severe head injuries whose
intracranial hypertension is refractory to maximal
medical and surgical ICP-lowering therapy. When
considering the institution of high-dose barbiturate
therapy, hemodynamic stabilization of the patient is
a prerequisite. The prophylactic use of barbiturate
coma is not indicated.
V. Anesthetic management
Anesthesia. The major goals of anesthetic management
are to (a) optimize cerebral perfusion and
oxygenation, (b) avoid secondary damage, and (c)
provide adequate surgical conditions for the
neurosurgeons. General anesthesia is recommended to
facilitate control of respiratory and circulatory
function.
Induction of anesthesia. Most patients who have
severe head injury have already had an endotracheal
tube inserted either during triage in the emergency
department or for their CT
examination. The patient who comes to the operating
room without endotracheal intubation is treated with
immediate oxygenation and securing of the airway.
Anesthesiologists must be aware that these patients
often have a full stomach, decreased intravascular
volume, and a potential cervical spine injury.
Direct arterial pressure monitoring by an indwelling
arterial catheter inserted before the induction of
anesthesia is recommended. Either the radial artery
or the dorsalis pedis artery may be cannulated,
depending on other sites of injury.
Several induction techniques are recommended. The
patient's presentation and hemo-dynamic stability
determine the choice.
Rapid sequence induction may be desirable in
hemodynamically stable patients, although this
procedure can produce an elevation in blood pressure
and ICP. During administration of 100% oxygen, an
induction dose of thiopental, 3 to 4 mg/kg, or
propofol, 1 to 2 mg/kg, and succinylcholine, 1.5
mg/kg, is administered and the trachea is intubated.
Etomidate, 0.2 to 0.3 mg/kg, may be administered in
patients in whom the circulatory status is
concerning. In hemodynamically unstable patients,
the dose of induction drugs is substantially
decreased or even omitted. However, cardiovascular
depression is always a concern, especially in
hypovolemic patients.
Succinylcholine has been shown to increase ICP. The
prior administration of small doses of a
nondepolarizing muscle relaxant may prevent this
increase but not predictably. Succinylcholine
remains a good choice, however, to facilitate rapid
laryngoscopy and to secure the airway. Rocuronium,
0.6 to 1 mg/kg, is an excellent alternative because
of its rapid onset of action and lack of effect on
intracranial dynamics.
Intravenous induction. When the patient is stable
and does not have a full stomach, anesthesia can be
induced by titrating the dose of either thiopental
or propofol to minimize circulatory instability. An intubating dose of a nondepolarizing muscle relaxant
is given with or without priming to facilitate
intubation within a short period of time. For
example, rocuronium, 0.6 to 1 mg/kg, allows
satisfactory intubating conditions within 60 to 90
seconds. Fentanyl, 1 to 4 mcg/kg i.v.,
is administered to blunt the hemodynamic response to
laryngoscopy and intubation. Lidocaine, 1.5 mg/kg
i.v., given 90 seconds before laryngoscopy, can help
prevent the increase in ICP.
A large-bore oral gastric tube is inserted after
intubation, and gastric contents are initially
aspirated and then passively drained during the
operation. Nasal gastric tubes are avoided because
of the potential presence of a basilar skull
fracture.
Maintenance of anesthesia. The ideal drug for
maintenance of anesthesia should reduce ICP,
maintain adequate oxygen supply to the brain tissue,
and protect the brain against ischemic-metabolic
insult. No gold-standard anesthetic drug fulfills
these requirements for head injury. The selection of
anesthetic drugs is based on a consideration of the
intracranial pathology as well as systemic
conditions such as cardiopulmonary disturbances and
the presence of multisystem trauma.
Anesthetics
(1) Intravenous anesthetics
(a) Barbiturates. Thiopental and pentobarbital
decrease CBF, cerebral blood volume (CBV), and ICP.
The reduction in ICP with these drugs is related to
the reduction in CBF and CBV coupled with metabolic
depression. These drugs will also have these effects
in patients who have impaired CO2 response.
Thiopental and pentobarbital have been shown in
animal models to protect against focal brain
ischemia. In head injury, ischemia is a common
sequela. Although barbiturates might be effective in
head injury, no prospective, randomized clinical
trial has demonstrated that they definitely improve
outcome after TBI. In addition, barbiturates can be
detrimental in patients with head injuries because
of their cardiovascular-depressant effect. Also,
when barbiturates are administered for prolonged
periods, their duration of action is increased.
(b) Etomidate. As with barbiturates, etomidate
reduces CBF, CMRo2, and ICP. Systemic hypotension
occurs less frequently than with barbiturates.
Prolonged use of etomidate may suppress the
adrenocortical response to stress.
(c) Propofol. The cerebral hemodynamic and metabolic
effects of propofol are similar to those of
barbiturates. Propofol might be useful in patients
who have intracranial pathology if hypotension is
avoided. Because the context-sensitive half-life is
short, emergence from anesthesia is rapid, even
after prolonged administration. This may offer an
advantage over other intravenous anesthetics in
providing the opportunity for early postoperative
neurologic evaluation. Because of propofol's potent
circulatory depressant effect, however, meticulous
care should be exercised to maintain adequate CPP,
including the correction of hypovolemia prior to
administering propofol. Recent studies have shown a
reduction in jugular bulb oxygen saturation during
propofol anesthesia. Propofol can also reduce CBF
more than CMRo2, producing ischemia under certain
conditions. Therefore, care should be taken when
hyperventilating patients during propofol
anesthesia.
(d) Benzodiazepines. Diazepam and midazolam may be
useful either for sedating patients or inducting
anesthesia because these drugs have minimal
hemodynamic effects and are less likely to impair
cerebral circulation. Diazepam, 0.1 to 0.2 mg/kg,
may be administered for inducting anesthesia and
repeated, if necessary, up to a total dose of 0.3 to
0.6 mg/kg. Midazolam, 0.2 mg/kg, can be used for
induction and repeated as necessary.
(e) Narcotics. In clinical doses, narcotics produce
a minimal to moderate decrease in CBF and CMRo2.
When ventilation is adequately maintained, narcotics
probably have minimal effects on ICP. Despite its
small ICP-elevating effect, fentanyl provides
satisfactory analgesia and permits the use of lower
concentrations of inhalational anesthetics. Some
reports have shown that sufentanil increases ICP in
patients with severe head injuries. This could
result from the autoregulatory response (i.e.,
cerebral vasodilatation) to the sudden decrease in
systemic blood pressure. When these drugs are used,
measures to maintain systemic blood pressure need to
be implemented.
(2) Inhalational anesthetics
(a) Isoflurane. A potent metabolic depressant,
isoflurane has less effect on CBF and ICP than
halothane has. Because isoflurane depresses cerebral
metabolism, it may have a cerebral protective effect
when the ischemic insult is not severe. Data favor
the use of isoflurane over either halothane or
enflurane. Isoflurane in concentrations of >1
minimum alveolar concentration should be avoided,
however, because it can cause substantial increases
in ICP.
(b) Sevoflurane. In the rabbit cryogenic
brain-injury model, the elevation of ICP occurring
in association with an elevation in blood pressure
was higher in the animals anesthetized with
sevoflurane than with halothane. Clinical studies
have demonstrated, however, that sevoflurane's
effect on cerebral hemodynamics is either similar to
or milder than that of isoflurane. The disadvantage
of sevoflurane is that its biodegraded metabolite
may be toxic in high concentrations. There is no
evidence of an adverse effect at clinically used
concentrations, however, unless sevoflurane is
administered in a low-flow circuit for prolonged
periods. Rapid emergence from anesthesia with
sevoflurane may be an advantage because it
facilitates early postoperative neurologic
evaluation.
(c) Desflurane. Desflurane at high concentrations
appears to increase ICP.
(d) Nitrous oxide (N2O). N2O dilates cerebral
vessels, thereby increasing ICP. Patients who have
intracranial hypertension or a decrease in
intracranial compliance should, therefore, not
receive this drug. N2O should also be avoided in the
presence of pneumocephalus or pneumothorax because
it diffuses into an airspace more rapidly than the
nitrogen diffuses out, thereby increasing the volume
within the airspace.
(3) Local anesthetic. The infiltration of either
lidocaine 1% or bupivacaine 0.25%, with or without
epinephrine, in the skin around the scalp incision
and the insertion sites for the pin head holder is
helpful in preventing systemic and intracranial
hypertension in response to these stimuli and
avoiding the unnecessary use of deep anesthesia.
(4) Muscle relaxants. Adequate muscle relaxation
facilitates appropriate mechanical ventilation and
reduces ICP. Coughing and straining are avoided
because both can produce cerebral venous
engorgement.
(a) Vecuronium appears to have minimal or no effect
on ICP, blood pressure, or heart rate and would be
effective in patients with head injuries. This drug
is given as an initial dose of 0.08 to 0.1 mg/kg
followed by infusion at a rate of 1 to 1.7
mcg/kg/minute.
(b) Pancuronium does not produce an increase in ICP
but can cause hypertension and tachycardia because
of its vagolytic effect, thereby increasing the
patient's risk.
(c) Atracurium has no effect on ICP. Because of its
rapid onset and short duration of action, a bolus
dose of 0.5 to 0.6 mg/kg followed by a continuous
infusion at a rate of 4 to 10 mcg/kg/minute is
administered with monitoring of neuromuscular
blockade.
(d) Rocuronium is useful for intubation because of
its rapid onset of action and lack of effect on
intracranial dynamics. For maintenance, drugs with
longer durations of action are recommended.
Intraoperative respiratory and circulatory
management
Mechanical ventilation. Mechanical ventilation is
adjusted to maintain a Paco2 of around 35 mm Hg. The
fraction of inspired oxygen (Fio2) is adjusted to
maintain a Pao2 of >100 mm Hg.
Patients, especially those who have pulmonary
contusion, aspiration, or central neurogenic
pulmonary edema, may require positive end-expiratory
pressure (PEEP) to maintain adequate oxygenation.
Excessive PEEP should be avoided, however, because
the elevation in intrathoracic pressure can
compromise cerebral venous drainage and increase
ICP.
Circulatory management. CPP should be maintained
between 60 and 110 mm Hg. The transducer for direct
monitoring of arterial blood pressure is zeroed at
the level of mastoids to reflect the cerebral
circulation.
When hypotension persists despite adequate
oxygenation, ventilation, and fluid replacement,
careful elevation of the blood pressure with a
continuous infusion of an inotrope or vasopressor
may be necessary. Phenylephrine, 0.1 to 0.5
mcg/kg/minute, and dopamine, 1 to 10 mcg/kg/minute,
are appropriate drugs in this setting. A bolus dose
of vasopressor must be used cautiously because
abrupt increases in blood pressure can elevate ICP
to dangerous levels, especially in patients who have
disordered autoregulation.
Hypertension is treated cautiously because the
elevation in blood pressure may reflect compensatory
hyperactivity of the sympathetic nervous system in
response to elevated ICP and compression of the
brain stem (Cushing's reflex). Adequate oxygenation,
ventilation, volume replacement, and analgesia
should be first assessed and corrected. When
necessary, an antihypertensive drug, such as either
labetalol or esmolol, which has minimal cerebral
vasodilating effects, should be administered. When
treating hypertension, maintenance of CPP is a major
concern.
Intraoperative management of elevated ICP
Patient's posture. A slight head-up tilt of 10° to
30° is desirable. CPP might not be improved,
however, if systemic blood pressure decreases
substantially. When the surgeon requests either
rotation or flexion of the head and the neck, the
anesthesiologist must ensure the adequacy of venous
return.
Ventilation. The Paco2 is maintained at around 35 mm
Hg. Hyperventilation is best avoided unless
monitoring ensures adequate brain oxygenation.
Circulation. Both hypotension (systolic blood
pressure of <90 mm Hg) and hypertension (systolic
blood pressure of >160 mm Hg) should be corrected
when indicated.
Diuretics
(1) Mannitol decreases cerebral volume and reduces
ICP.
(2) Furosemide may be coadministered in severe cases
as well as in the patient who has compromised
cardiac function and the potential for heart
failure. Furosemide, 0.1 to 0.2 mg/kg, is given 15
minutes before mannitol administration. When
furosemide and mannitol are administered, careful
monitoring of intravascular volume either by CVP or
pulmonary artery pressure is necessary.
Ventilation, oxygenation, depth of anesthesia, and
last dose of diuretics should be assessed in the
patient if protrusion of the brain is observed after
craniotomy. If all are adequate, additional
thiopental (or pentobarbital) may be indicated. More
vigorous hyperventilation is also an option with
careful monitoring of brain oxygenation. If these
measures fail, decompressive craniectomy may be
necessary.
CSF drainage. If an intraventricular catheter is in
place, CSF drainage is an effective and reliable
technique for reducing ICP.
Monitoring
Standard monitoring includes heart rate and rhythm
(electrocardiogram), noninvasive and direct arterial
blood pressure measurement, pulse oximetry,
end-tidal CO2, body temperature, urinary output, CVP,
and neuromuscular blockade. Arterial blood gases,
hematocrit, electrolytes, glucose, and serum
osmolarity should be measured periodically.
Monitoring for air embolism. Detection of venous air
embolism by Doppler ultrasound should be considered
for surgical procedures in which veins in the
operative site are above the level of the heart.
Brain monitoring as with an electroencephalogram,
evoked potentials, jugular venous bulb oxygen
saturation (Sjo2), flow velocity measured by
transcranial Doppler (TCD), brain tissue Po2
(btPo2), and ICP may be used.
1. Sjo2. The Sjo2 provides continuous information about
the balance between global cerebral oxygen supply
and demand. An Sjo2 of <50% for >15 minutes is a
poor prognostic sign and is often associated with a
poor neurologic outcome. The decrease in Sjo2 could
be caused by excessive hyperventilation, decreased
CPP, cerebral vasospasm, or a combination. The major
causes of a decrease in Sjo2 and their treatment are
listed in Table -2.
2. Flow velocity of basal cerebral arteries as measured
by the TCD technique is helpful in assessing the
cerebral circulatory state at the bedside. However,
it does not provide an absolute value for the CBF.
High-normal values may indicate hyperemia or
vasospasm. The TCD waveform can differentiate
between these two conditions. A disadvantage of this
monitor is that the application of the Doppler probe
is not always possible during the surgical
procedure.
3. Near-infrared spectroscopy, currently available in
clinical practice, provides relative information
about changes of oxy- and deoxyhemoglobin and the
cytochrome oxidase redox status in the brain tissue
of interest in a noninvasive and continuous fashion.
4. ICP. The association between severity of ICP
elevation and poor outcome is well known. Monitoring
ICP is useful, therefore, not only as a guide to
therapy, but also for assessing
the response to the therapy and determining the
prognosis.
Table -2. Major causes of decreased Sjo2a and
treatment |
Cause |
Clinical condition |
Treatment
|
Cao2 |
Hypoxemia |
Correction of hypoxemia |
Anemia |
Blood transfusion
|
CBF |
Hypotension |
Fluid replacement; inotropics
and vasopressors |
Hyperventilation |
Correction of Paco2
|
Intracranial
hypertension |
Mannitol, furosemide,
barbiturate, propofol |
CMRo2 |
Hyperthermia |
Cooling |
Seizures |
Barbiturate, propofol |
Cao2, oxygen content in arterial blood; CBF,
cerebral blood flow; CMRo2, cerebral metabolic rate
for oxygen consumption. |
aSjo2 OC[Cao2-CMRo2/CBF]. |
5. btPo2. A probe for the determination of btPo2 is
available. A btPo2 of <10 mm Hg is assumed to convey
the risk of hypoxic injury. The disadvantages of
btPo2 monitoring include the facts that it (a) only
provides focal monitoring, (b) cannot be used in the
surgical field during operation, and (c) has a
critical threshold that is not well determined.
VI. Cerebral protection
Hypothermia. A reduction of body temperature to
33°C to 35°C may confer cerebral protection.
Protective mechanisms include a reduction in
metabolic demand, excitotoxicity, free radical
formation, and edema formation. In an animal
ischemia model, mild hypothermia of approximately
34°C to 36°C markedly attenuated ischemic injury.
In clinical practice, controversy concerning the
effectiveness of hypothermia in head injury still
continues. The multi-institutional study of
postoperative mild hypothermia in patients with head
injury was terminated by its Safety Monitoring Board
after enrolling 392 patients (see Clifton G et al.).
The results showed no difference in mortality
between patients with hypothermia and normothermia,
and patients with hypothermia experienced more
medical complications. Subgroup analysis revealed
that younger patients (45 years of age or younger)
who were hypothermic on admission and assigned to
the hypothermic group tended to have better outcomes
than those assigned to the normothermic group. A new
study of this group with an earlier induction of
hypothermia and more consistent critical care has
been initiated.
When induction of hypothermia is elected, meticulous
care is necessary to avoid adverse side effects such
as hypotension, cardiac arrhythmias, coagulopathies,
and infections. Rewarming should be carried out
slowly. Temperature monitoring at two or more sites
is recommended and may include the tympanic
membrane, nasopharyngeal area, esophagus, and blood.
VII. Postoperative management
Emergence and extubation. Anesthesiologists often
receive requests to awaken patients promptly to
allow early postoperative neurologic assessment.
Patients who had a normal level of consciousness
before the operation and who have undergone an
uneventful procedure can be awakened and their
tracheas extubated in the operating room, assuming
that emergence criteria have been satisfactorily
met. Smooth emergence with control of systemic blood
pressure and avoidance of coughing is necessary
to prevent postoperative cerebral edema and hematoma
formation.
Contraindications to extubation. Extubation in the
operating room is discouraged for patients whose
level of consciousness was depressed preoperatively
and in whom brain swelling is either marked during
operation or expected to occur postoperatively.
Patients who have sustained multiple traumatic
injuries are also candidates for postoperative
ventilation. Patients who are hypothermic during
emergence should be mechanically ventilated
postoperatively and their tracheas extubated after
careful rewarming.
VIII. Summary The major goal of perioperative management of
patients with head injuries is to prevent secondary
damage. Therapeutic measures based on established
guidelines and recommendations must be instituted
promptly and continued throughout the perioperative
course. Appropriate selection of anesthetics and
meticulous general management of respiration,
circulation, metabolism, fluid replacement, and
temperature are all essential to improve outcome.
|