| Head Injury |
Supratentorial Tumors |
Posterior Fossa Surgery|
Intracranial Aneurysms
|Ischemic Cerebrovascular Diseases
|Neuroendocrine Tumors
|Epilepsy-Awake
Craniotomy-Intraoperative MRI
|Spinal Cord Injury and Procedures
|Pediatric Neuroanesthesia
|Neurosurgery in the Pregnant
Patient
|Management of Therapeutic
Interventional Neuroradiology
|Management in Diagnostic
Neuroradiology
|
I. General considerations
Spinal anatomy and physiology
Structure
1.
The vertebral column consists of 33 superimposed
vertebrae that are divided into 7 cervical (C), 12
thoracic (T), 5 lumbar (L), 5 sacral (fused), and 4
coccygeal (fused) bones. Each individual vertebra
(Figure-1) is composed of a ventral vertebral
body and a dorsal bony neural arch formed from a
pedicle on either side of the vertebral body, both
of which join with the lamina posteriorly to form
the spinous process. The posterior vertebral body,
pedicles, and lamina form the vertebral foramen. The
neural laminar arches bear lateral transverse
processes and superior and inferior articular
facets.
2.
The vertebral column is stabilized (from posterior
to anterior) by the supraspinous, interspinal,
ligamentum flavum, posterior longitudinal, and
anterior longitudinal ligaments (Figure-1). The
ligaments provide flexibility and limit excessive
movement that could damage the spinal cord.
3.
The spinal cord begins at the foramen magnum and
terminates at the conus medullaris (L2 in adults).
Below the termination of the spinal cord, the lumbar
and sacral roots form the cauda equina. The spinal
cord is surrounded by the meninges, layers of tissue
including the dura mater and arachnoid membranes
which have cerebrospinal fluid (CSF) between them.
This provides additional protection for the spinal
cord. The anterior portion of the cord gives rise to
the motor nerves. Nerves that originate posteriorly
are sensory in function.
Blood supply
1.
Anterior spinal artery. A single vessel, formed from
the union of the two anterior spinal branches of the
vertebral arteries, descends down the entire length
of the anterior portion of the spinal cord. This
artery supplies perfusion to the anterior 75% of the
cord including the anterior column and most of the
lateral column.
Figure-1. A: View of a typical vertebra from above;
B: Lateral view; C: Ligaments of the spine. |
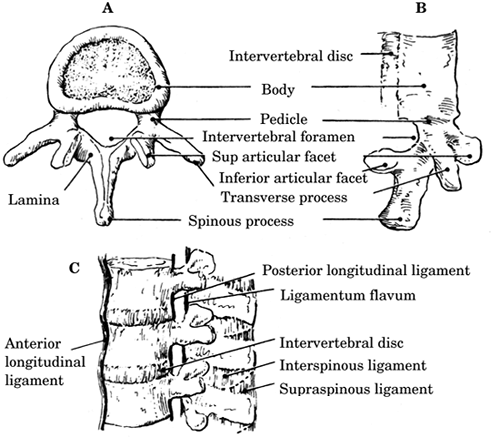 |
Posterior spinal arteries. Two vessels originating
from the posterior inferior cerebellar arteries
supply the posterior 25% of the cord including the
entire posterior column and the balance of the
lateral column.
Radicular arteries. These vessels originate from
branches of the vertebral, deep cervical, intercostal, and lumbar arteries. They anastomose
with the anterior and posterior spinal arteries. The
artery of Adamkiewicz (arteria radicularis magna),
the major radicular artery, is most commonly located
in the lower thoracic or upper lumbar region and
provides most of the blood supply to the lower cord.
Regulation of blood flow (Figure-2).
Autoregulation maintains spinal cord blood flow
(SCBF) by altering vascular resistance in response
to changes in mean arterial pressure (MAP).
1.
Normal autoregulation exists for a MAP between 50
and 150 mm Hg.
2.
Failure of autoregulation at a MAP of <50 mm Hg
leads to ischemia.
Figure -2.
Illustration of effect of changes in
Paco2, Pao2, and mean arterial pressure
(MAP) on spinal cord blood flow (SCBF) |
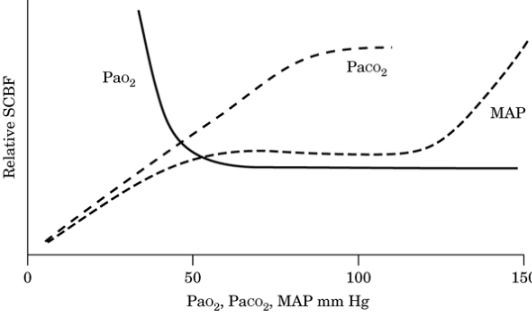 |
3.
At a MAP of >150 mm Hg, the increased flow leads to
tissue edema and disruption.
4.
The autoregulatory range of individuals who have
chronic hypertension is shifted to the right (higher
range of MAP).
5.
Alterations in the partial pressure of carbon
dioxide (Paco2) and oxygen (Pao2) disrupt
autoregulation in the spinal cord much as they do in
the brain. Between 20 and 80 mm Hg, SCBF is linearly
related to Paco2. SCBF is well maintained at varying
degrees of oxygenation until Pao2 falls to 50 mm Hg;
below this value, SCBF increases as oxygenation
decreases further.
II. Spinal cord injury (SCI)
Introduction. In the United States, 11,000 new cases
of SCI occur each year. The annual incidence of
traumatic SCI is approximately 40 cases per million
population, with an estimated prevalence approaching
250,000 cases. SCI in the United States remains more
common in males than in females by a 4:1 ratio.
Early mortality is around 50% with <10% of survivors
experiencing neurologic improvement. Accordingly,
the perioperative care of patients during the acute
phase of injury is extremely important.
Perioperative strategies that prevent further
injury, limit the extension of the existing injury,
or salvage even a few dermatomal levels can have a
significant influence on morbidity, mortality,
long-term disability, quality of life, and health
care costs.
The distribution of SCI consists of incomplete
tetraplegia, 29.5%; complete paraplegia, 27.9%;
incomplete paraplegia, 21.3%; and complete
tetraplegia, 18.5%. Common causes of SCI include
motor vehicle accidents (40% to 56%), falls (15% to
20%), violence (5% to 20%), sports injuries (5% to
16%), and other causes (5% to 10%). Most injuries
occur at the mid-cervical (C4-6, C5 most common) or
thoracolumbar (T12) region and are often associated
with concomitant injuries. Surgical treatment for
SCI is aimed at immobilization, medical
stabilization, spinal alignment, operative
decompression, and spinal stabilization. The focus
here is directed on the perioperative care of the
patient who has acute SCI or is at risk for it. It
also discusses specific issues pertaining to the
intermediate or chronic phase of injury.
Pathogenic correlates of SCI
Primary SCI, caused by the mechanical forces of the
trauma, results in direct neuronal disruption and
destruction, petechial hemorrhages, and hematomyelia.
Histologic changes consist of hemorrhage and protein
extravasation into the central gray matter, which
spread to the adjacent white matter. The traumatized
areas then undergo cavitating necrosis and
ultimately glial scar formation. Spinal cord edema
is maximal at 3 days and can persist for 2 weeks.
Rarely does physical transection of the spinal cord
occur.
Secondary SCI is caused by the activation of
biochemical, enzymatic, and microvascular processes
in proportion to the severity of the initial lesion.
Damage results from progressive hemorrhagic
necrosis, loss of cellular membrane integrity,
edema, inflammation, arachidonic acid release, lipid
peroxidation, and loss of vascular autoregulation.
These processes lead to vascular stasis, decreased
blood flow, ischemia, and cell death.
Anatomical correlates of SCI.
(Table-1) Flexion
injuries cause anterior subluxation or
fracture-dislocations of the vertebral bodies.
Hyperextension is associated with transverse
fractures of the vertebra, disruption of the
anterior longitudinal ligaments, and posterior
dislocations. Vertical compression produces burst
fractures and ligamentous rupture. Rotational
injuries result in fractures of the vertebral
peduncles and facets. The designation of a SCI as
stable or unstable considers the potential for
furthering spinal injury or failure to heal properly
if left untreated.
Table -1. Spinal injury, clinical finding, and
indicated treatment |
Spinal Injury |
Clinical Finding |
Treatment |
Atlanto-occipital
dislocation |
Usually unstable;
commonly fatal |
Reduction, immobilization, fusion |
Atlantoaxial injury
|
Isolated atlas
fracture |
Usually stable/no
neurologic injury |
Philadelphia collar |
Isolated odontoid
fracture |
Usually
neurologically intact |
Immobilization |
Displaced fracture
C1-2 |
Commonly fatal or
quadriplegic |
Immobilization and reduction |
Posterior subluxation
C1-2 |
Usually
neurologically intact |
Immobilization |
Axis pedicle
fracture |
Can be
neurologically intact |
Immobilization |
Hyperflexion
dislocation C3-T1 |
Any subluxation is
unstable |
If neurologic deficit, decompression |
Dislocated facets |
Neurologically
variable |
Traction
and surgery |
Flexion-rotation
injuries |
Neurologically
variable |
Surgical reduction and fusion if anterior subluxation and jumped facet |
Compression fractures C3-T1 |
Wedge
compression/burst fractures |
Frequent neurologic
damage |
Surgical decompression |
Teardrop fractures
(vertebra dislocated anteriorly with
inferior vertebral fracture) |
Usually unstable |
Posterior fusion
|
Hyperextension
injuries |
Geriatric patients with spondylosis
producing central cord syndrome |
Immobilization; if significant spinal canal
narrowing, decompression |
Thoracic spine
injuries |
Incomplete
neurologic injury most common |
Realignment and stabilization
|
Thoracolumbar
injuries |
Neurologic deficits
complex |
Decompression and fusion |
Lumbar injuries |
Incomplete
neurologic injury |
Realignment and decompression |
Penetrating
injuries |
Neurologic deficit
variable |
Decompression/foreign body removal
|
Clinical correlates of SCI. SCI can result in either
complete (total loss of sensory and motor function
distal to the injury) or incomplete (presence of any nonreflex function distal to the injury;
Table-2) loss of neurologic function. Complete SCI has
less than a 10% chance of total return of normal
neurologic function. Incomplete SCI has a 59% to 75%
chance of recovering
lost function. Table-3 details the range of
cardiac and respiratory dysfunction, depending on
the site of acute SCI. Medical problems include
these:
Cardiovascular
1.
Spinal shock
2.
Bradycardia
3. Myocardial contractility
4.
Deep venous thrombosis
5.
Hypothermia
Respiratory
1.
Respiratory impairment
2.
Poor cough
3.
Viscous mucous
Gastrointestinal
1.
Atony
2.
Prone to aspiration
Genitourinary
1.
Bladder distension
2.
Infection
Electrolytes
1.
Hypercalcemia
2.
Hyperphosphatemia
3.
Hyponatremia
4.
Hyperkalemia
Table-2. Incomplete spinal cord injury syndromes
|
Syndrome |
Clinical Findings
|
Anterior cord
syndrome |
Motor, sensory, temperature
and pain lost; vibration/position intact |
Central cord
syndrome |
Motor impairment of upper more
than lower extremities |
Posterior cord
syndrome |
Loss of fine, vibratory, and
position sensation; preserved motor function |
Brown-Sequard (hemicord)
syndrome |
Ipsilateral
paralysis, loss of proprioception, touch, and
vibration; contralateral loss of pain and
temperature |
Conus medullaris syndrome |
Areflexic bladder, bowel,
and lower extremities; sacral reflexes can be
preserved; reduced rectal tone and perirectal
sensation |
Cauda equina
syndrome |
Sensory loss with flaccid
weakness; sacral reflexes abnormal or absent |
The effect of SCI on the cardiovascular system
depends on the level of injury (Table-3). For
levels of SCI below T6, the major problem involves
varying degrees of hypotension resulting from the
functional sympathectomy. With complete SCI above
T6, more significant cardiovascular abnormalities
including bradycardia, hypotension, ventricular
dysfunction, and dysrhythmias are encountered.
Spinal shock, seen most commonly with physiologic or
anatomic transection of the spinal cord
above C7, results from a total loss of impulses from
higher centers as an immediate consequence of the
injury.
Table-3. Level of spinal cord injury and
pulmonary/cardiac function |
Level of Spinal Cord Injury |
Pulmonary Function Ventilatory Function |
Cough |
Cardiovascular Function Sympathetic Function |
Cardiovascular Reserve |
C1-2 |
0 |
0 |
Minimal |
Minimal |
C3-4
|
0 |
0 |
Minimal |
Minimal |
C5-6 |
+ |
+ |
Minimal |
Minimal |
C7 |
+ to
++ |
+ to
++ |
Minimal |
+ |
High
thoracic |
++ |
++ |
+ to
++ |
++ |
Low
thoracic |
++
to +++ |
++
to +++ |
++
to +++ |
++ to +++ |
Lumbar |
+++ |
+++ |
+++ |
+++ |
Sacral |
+++ |
+++
|
+++ |
+++
|
Scale is 0 (no function) to +++ (normal).
|
1.
Spinal shock is characterized by flaccid paralysis,
loss of reflexes below the level of the lesion,
paralytic ileus, and loss of visceral and somatic
sensation, vascular tone, and the vasopressor
reflex.
2.
This syndrome of autonomic dysfunction and loss of
sensory and motor function lasts from days to weeks
and is prolonged by serious infection.
3.
Neurogenic shock is manifest by hypotension,
bradycardia, and hypothermia. The higher the spinal
level of injury, the more severe the physiologic
derangements. Shock occurs from the disruption of
the sympathetic outflow from T1-L2, which results in
unopposed vagal tone and vasodilatation with pooling
of blood in the peripheral vascular beds. The most
common cardiovascular abnormalities encountered
after an acute cervical SCI and the associated
incidence are listed in the following table.
Tabel-4. Cardiovascular
derangement in neurogenic shock |
Marked bradycardia
(<45 beats/minute) |
71% |
Episodic
hypotension |
68% |
Need for intravenous pressors |
35% |
Use of atropine or temporary transvenous
pacemaker |
29% |
Primary cardiac
arrest |
16% |
These cardiovascular derangements remain most
problematic during the first 2 weeks after acute
cervical SCI.
(1) Bradycardia, universal with acute complete
cervical SCI, results from a functional sympathectomy with interruption of cardiac
accelerator nerves (T1-4) and unopposed vagal
innervation. Bradycardia usually resolves over a 2-
to 6-week period. More profound degrees of
bradycardia, as well as cardiac arrest, can occur
during stimulation of the patient (e.g., turning the
patient, performing tracheal suctioning).
Familiarity with the factors precipitating
bradycardia lead to the use of preventive
interventions (sedation, anticholinergics, 100%
oxygen before suctioning, and limiting the time
allowed for suctioning). Although the bradycardia is
effectively treated with atropine in most cases, a
temporary pacemaker can be required.
(2) Hypotension, defined as a systolic blood
pressure (BP) below 90 mm Hg or 30%
below baseline, is seen in 60% to 80% of patients
after acute cervical SCI. Early intervention to
maintain the MAP at 85 mm Hg for the first 7 days
after injury is recommended to preserve neurologic
function while autoregulation is impaired. The heart
rate is useful in differentiating between neurogenic
shock, manifest by the triad of bradycardia,
hypotension, and hypothermia, and hemorrhagic shock
as the cause of tachycardia. Hypovolemia from
coexisting hemorrhagic shock in patients who also
have spinal shock is treated with prompt blood
replacement and administration of isotonic
crystalloid. The total volume of fluid administered
is limited because pulmonary edema and cardiac
decompensation can occur, especially in the setting
of high SCI.
(a) If hypotension persists despite adequate fluid
administration, vasopressor therapy should be
instituted. The vasopressor should have beta-agonist
properties (e.g., dopamine or dobutamine).
Supplementation with an alpha-agonist such as
phenylephrine could be necessary. However, care is
indicated when choosing a more potent alpha-agonist,
such as norepinephrine, which can substantially
increase cardiac afterload, impair cardiac output,
and precipitate frank left ventricular failure.
(b) Invasive central hemodynamic monitoring is
recommended in high SCI as an aid in guiding the
clinical management of hypotension. A pulmonary
artery occlusion pressure of 14 to 18 mm Hg appears
to optimize spinal cord perfusion. In patients who
have suffered multiple trauma, hypotension and
bradycardia secondary to neurogenic shock can
conceal hemorrhagic shock. Operative intervention
for spinal injury should be postponed until the
patient's hemodynamic status has been optimized.
Disturbances of cardiac rhythm are commonly observed
in SCI and include bradycardia, primary asystole, supraventricular dysrhythmias (atrial fibrillation,
reentry supraventricular tachycardia), and
ventricular dysrhythmias. An acute autonomic
imbalance resulting from a disruption
of sympathetic pathways in the cervical cord is
causative. The arrhythmias usually resolve within 14
days of injury.
Left ventricular impairment has been noted in
complete cervical SCI and is attributed to the
functional sympathectomy with resulting autonomic
imbalance.
Autonomic hyperreflexia occurs in 85% of patients
who have spinal cord transections above T6. This
clinical constellation is secondary to autonomic
vascular reflexes, which usually begin to appear
approximately 1 to 3 weeks after injury (when spinal
shock has resolved).
1.
Afferent impulses originating from cutaneous,
proprioceptive, and visceral stimuli (bladder or
bowel distention, childbirth, manipulations of the
urinary tract, or surgical stimulation) are
transmitted to the isolated spinal cord. They, in
turn, elicit a massive sympathetic response from the
adrenal medulla and sympathetic nervous system,
which is no longer modulated by the normal
inhibitory impulses from the brain stem and
hypothalamus. Vasoconstriction occurs below the
level of the spinal cord lesion. Reflex activity of
carotid and aortic baroreceptors produces
vasodilatation above the lesion, which is often
accompanied by bradycardia, ventricular
dysrhythmias, and even complete heart block.
2.
Common signs and symptoms include hypertension,
bradycardia, hyperreflexia, muscle rigidity and
spasticity, diaphoresis, pallor, flushing above the
lesion, and headache. Horner's syndrome, pupillary
changes, anxiety, and nausea occur less frequently.
Systolic pressures in excess of 260 mm Hg and
diastolic pressures of 220 mm Hg have been reported.
3.
Adverse sequelae include myocardial ischemia,
intracranial hemorrhage, pulmonary edema, seizures,
coma, and death.
4. Treatment involves cessation of the offending
stimulus and a change to the upright position
(pooling of blood in the lower extremities).
Pharmacologic intervention includes direct-acting
vasodilators (e.g., sodium nitroprusside),
beta-blocking drugs (e.g., esmolol), combination
alpha- and beta-blocking drugs (e.g., labetalol),
calcium-channel blocking drugs, and ganglionic
blocking drugs. Because the attacks are often
paroxysmal, drugs of rapid onset and short duration
are preferred.
Acute care of the patient after SCI. Preservation of
spinal cord function involves maintaining oxygen
delivery, stabilizing the spine, and decreasing
spinal cord
edema and the secondary biochemical processes that
exacerbate the neurologic injury. The initial care
of the patient after SCI can be considered in the
following areas:
External splinting and immobilization. The spine is
immobilized in the field by placing the patient on a
spine board with sandbags on either side of the head
to prevent rotation.
Medical management. Identification of associated
injuries is crucial. These include the following:
1.
Cervical Spine Injury
a.
Head
b.
Airway
c.
Esophagus
2.
Lumbar Spine Injury
a.
Abdominal
b.
Pelvic
3.
Thoracic Spine Injury
a.
Myocardial
b.
Pulmonary
c.
Ribs
d.
Major vascular
Airway
(1) SCI presents several problems in airway
management. Many maneuvers used for intubation can
cause displacement and worsening of the injury,
especially at the level of the cervical spine
(C-spine). For this reason, all patients who have
head trauma, multiple trauma, or decreased level of
consciousness should be considered as having a
spinal injury until proved otherwise
radiographically (see Figure -3 for the algorithm
for airway management for suspected C-spine injury).
(2) Patients who have a normal level of
consciousness, an intact "gag reflex" and a
patent airway can be managed conservatively with
supplemental oxygen. However, these patients should
be observed closely to detect progressive loss of
ventilatory ability as a result of developing
diaphragmatic or intercostal paralysis. Because
profound reductions in forced vital capacity (FVC)
and expiratory flow rates are observed immediately
after injury, nearly all patients who have an acute
cervical SCI require mechanical ventilation within
24 to 48 hours of hospital admission.
(3) The initial airway management of the C-spine
injured patient includes consideration of the
following issues:
(a) The urgency of airway intervention
(b) The presence of associated facial, neck, or soft
tissue injuries
Figure-3. Airway management algorithm for the
patient who has suspected C-spine injury.
|
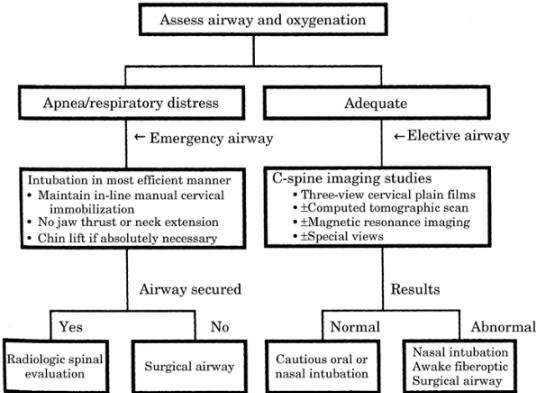 |
(c) The presence of basilar skull fracture or
midface fractures that contraindicate nasal
intubation
(d) The status of the patient, whether awake or not
(e) The skill of the operator in using different
airway techniques
(4) Direct laryngoscopy remains the method of choice
for emergent airway control in patients who have
confirmed or possible C-spine injuries. Mouth
opening for laryngoscopy can be facilitated by
removing the cervical collar, and neck movement can
be minimized by manual in-line stabilization without
axial traction. Manual in-line stabilization can in
fact result in more movement of unstable structures
lower in the neck. Manual in-line stabilization can
sometimes make a difficult intubation more
difficult. Cricoid pressure can be utilized to
reduce the risk of pulmonary aspiration of gastric
contents.
(5) Blind nasal intubation is commonly recommended
for airway management. The benefit of this technique
is minimal head movement. Potential disadvantages
include an extended period
of time to perform and trauma to the nasal passage.
Nasotracheal intubation is contraindicated in the
presence of a basilar skull fracture or extensive
facial trauma.
(6) Fiberoptic intubation is appropriate in
nonemergent situations in which the airway is free
of blood and stomach contents. This technique can
facilitate intubation of the larynx with the least
amount of neck movement.
(7) In patients who have particularly difficult
airways, a catheter passed through a needle inserted
into the cricothyroid membrane ("retrograde
intubation") and directed out the nose or mouth
can also serve as a guide for intubation. Cricothyroidotomy is also an appropriate choice for
an anatomically abnormal airway in an emergent
situation.
(8) Flexion and extension of the neck during
maintenance of the airway and intubation could lead
to catastrophic exacerbation of the SCI. Therefore,
in-line manual stabilization should be carefully
utilized when traditional laryngoscopy proves
necessary. This is accomplished by having an
assistant hold the sides of the neck and the mastoid
process, preventing any movement of the neck. The
airway can be opened with a "jaw thrust"
technique while the head is maintained in a neutral
position without either flexion or extension.
(9) Because of gastric atony and paralytic ileus,
SCI patients are considered to have "full
stomachs" and are at increased risk for
aspiration. Therefore, airway adjuncts such as the
laryngeal mask, although helpful in maintaining
oxygenation in a situation in which intubation has
proven difficult, should not be relied on as a
long-term solution. Cricoid pressure is administered
with minimum force to avoid inadvertent further
injury to the cord. As the application of cricoid
pressure can cause neck displacement of up to 9 mm,
the risk of causing or exacerbating C-spine injury
should be weighed against the risk of pulmonary
aspiration. Although excessive movement of the spine
is to be avoided, hypoxia secondary to a failure to intubate worsens the prognosis even more so that
intubation should be
accomplished as expeditiously as possible.
(10) A brief neurologic examination after intubation
reveals any further deterioration in the patient's
neurologic condition related to manipulation of the
airway or positioning in preparation for intubation.
Pulmonary system. SCI can have a profound effect on
the respiratory system, depending on the level and
degree of injury, because of paralysis of the
abdominal, intercostal, diaphragmatic, and accessory
muscles. Problems include respiratory failure,
recurrent lobar atelectasis, hypoventilation,
ventilation-perfusion mismatching, pulmonary edema
(neurogenic, cardiogenic), bacterial pneumonia,
aspiration pneumonitis, and coexisting blunt chest
trauma.
(1) Respiratory failure is present in nearly all
patients with SCI above C7. The resultant
abnormality in respiratory mechanics leads to a
reduction in lung volume (tidal volume, expiratory
reserve volume, functional residual capacity),
impairment of respiratory function (decreased FVC,
forced expiratory volume in 1 second [FEV1], peak
forces, peak flows), and retention of pulmonary
secretions with progressive hypoxemia and CO2
retention. In addition, patients can have abdominal
distention secondary to gastric atony, which further
impairs pulmonary function. Even patients whose
weakness does not extend above the abdominal muscles
are at risk for abnormal respiratory function
because of their inability to clear secretions.
(2) Patients require frequent assessment of
respiratory function because significant declines in
pulmonary reserve can occur before overt clinical
signs of respiratory failure are seen. It is
particularly important to perform serial
measurements of vital capacity (VC) and negative
inspiratory force. When the VC decreases to <50% of
predicted, more frequent serial determinations of VC
must be made (i.e., every 6 hours). When the VC
decreases to <1 L, especially if the patient is
dyspneic and hypoxemic, endotracheal intubation
should be performed.
(3) The aim of mechanical ventilation in this
scenario is to incorporate spontaneous patient
effort into the respiratory dynamic
so as to maintain diaphragmatic muscle mass. The
combination of synchronized intermittent mandatory
ventilation with pressure support will achieve this
goal.
(a) Positive end-expiratory pressure is added,
beginning with 5 cm H2O, to recruit collapsed
alveoli and prevent further atelectasis. Choosing
intermittent "sigh" breaths (e.g., 1 to 2 "sigh"
breaths per minute at 10 mL/kg) can also enhance
alveolar recruitment.
(b) Weaning from mechanical ventilation after
cervical SCI is facilitated by the respiratory
muscles' development of spasticity at approximately
3 weeks. Spasticity of the respiratory muscles
stabilizes the chest wall sufficiently to improve
lung volume and overall ventilatory ability. The VC
doubles in volume within 5 weeks of injury in
patients whose injuries are at the C4-5 level.
Respiratory dynamics in the supine position improve
owing to the more cephalad position of the
diaphragm. This position is therefore used when
weaning.
(4) Lobar atelectasis, common in SCI above C7,
requires aggressive pulmonary therapy emphasizing
the prevention, recognition, and treatment of
secretion retention. Instituted at the time of
hospitalization, this regimen includes frequent
nasotracheal suctioning, frequent repositioning or
rotational beds (i.e., continuous lateral rotation
to 45), chest percussion, bronchodilator
therapy, deep breathing exercises, incentive
spirometry, and assisted coughing. Even with the
most aggressive pulmonary care and management,
retained secretions result in repeated lobar
collapse during the first 2 weeks of
hospitalization. Therapeutic bronchoscopy is
frequently required during this period.
(5) Pulmonary edema is observed in patients who have
acute SCI. Neurogenic causes include intracranial
hypertension with high cervical cord injury and
increases in extravascular lung water secondary to
autonomic dysfunction with sympathetic discharge at
the time of injury. Cardiogenic pulmonary edema
could also occur because of reduced myocardial
inotropy and overzealous fluid administration.
Meticulous fluid management guided by central
monitoring is essential in limiting pulmonary
complications.
(6) Pneumonia is observed in 70% of cervical and
high thoracic spinal cord injuries. Pneumonia can
develop from aspiration of gastric contents at the
time of the initial injury or can occur later from
nosocomial bacterial infection.
(7) Chest trauma resulting in hemothorax, pulmonary
contusions, pneumothorax, and rib fractures could be
present in patients who have sustained SCI. These
injuries often necessitate prolonged mechanical
ventilation with difficulty in weaning and delayed
operative intervention to stabilize the spine.
Cardiovascular support. For the first few minutes
after SCI, a brief and substantial autonomic
discharge from direct compression of sympathetic
nerves occurs. This results in severe hypertension
and arrhythmias and can cause left ventricular
failure, myocardial infarction, and pulmonary
capillary leak. This transient phase is usually no
longer evident by the time the patient reaches the
hospital when hypotension from neurogenic shock and
traumatic hypovolemia is commonly seen.
Gastrointestinal (GI) tract. During the acute stages
of SCI, the GI tract loses autonomic neural input
and becomes atonic. Intestinal ileus (especially
after thoracic and lumbar SCI) and gastric atony can
cause gastric distention and place the patient at
risk for aspiration. Moreover, the dilatation can
also cause upward pressure on the diaphragm,
adversely affecting ventilation. Insertion of a
nasogastric tube limits distention and reduces the
risk of regurgitation.
(1) Because hypochloremic metabolic alkalosis can
occur with excessive gastric suctioning, careful
monitoring of fluid and electrolyte balance must be
provided.
(2) Gastritis and gastric ulceration and hemorrhage
can occur after SCI, especially in patients
requiring mechanical ventilation or as a result of
administration of corticosteroids. Preventive
techniques include monitoring gastric pH and the use
of antacids or H2 blocking drugs.
(3) Other diseases occurring in critically ill
patients after SCI include pancreatitis, acalculous
cholecystitis, and gastric perforation (especially
in those receiving high-dose steroids).
(4) Patients who have acute SCI are
characteristically catabolic. Therefore, the
initiation of nutritional supplementation within the
first few days after injury is advised.
Genitourinary. During the acute stages of SCI, the
bladder is flaccid. Insertion of a latex-free (to
prevent the development of latex allergy) indwelling
urinary catheter is often necessary. Adequate
hydration is likely if urinary volume is >0.5
mL/kg/hour in the absence of renal dysfunction.
(1) Bladder flaccidity is followed by bladder
spasticity. The abnormalities of bladder emptying
predispose the patient to recurrent urinary tract
infections, bladder stones, nephrocalcinosis, and
recurrent urosepsis.
(2) Although an indwelling drainage catheter is
required during the initial 2 to 3 weeks after
injury to prevent urinary retention and reflex vagal
responses, intermittent straight catheterization of
the bladder with latex-free catheters should be
instituted as soon as feasible.
(3) Acute renal failure is uncommon but can occur as
a result of hypotension, dehydration, sepsis, or
associated trauma.
Temperature control. The body temperature of
patients who have injuries above C7 tends to
approach that of the environment (poikilothermia)
owing to the inability to conserve heat in cold
environments through vasoconstriction and the
inability to sweat in hot ambient conditions.
Consequently, these patients are prone to
hypothermia if the ambient temperature is lower than
normal body temperature. While hypothermia can
provide some degree of spinal cord protection, it
can also increase the incidence of arrhythmias and
prolong the effects of anesthesia. Delayed awakening
is problematic because it interferes with prompt
neurologic examination.
Deep venous thrombosis (DVT). Without prophylaxis
against DVT after acute SCI, patients have an
incidence of asymptomatic DVT of 60% to 100%.
Venograms become positive within 6 to 8 days after
injury. Despite an increased awareness of DVT as a
complication of SCI, pulmonary embolism (PE) occurs
in 10% to 13% of SCI patients and ranks as the third
leading cause of death. Given the high risk of DVT
and PE after SCI, a multimodal prophylactic medical
management plan is recommended. With effective
treatment, the
occurrence of DVT can be decreased to 5%. A recent
consensus statement on the prevention of DVT in the
SCI patient has been published to guide therapy. It
includes the following:
(1) Thromboprophylaxis should be provided for all
patients after acute SCI.
(2) Single prophylactic modalities such as low-dose
unfractionated heparin (LDUH), graduated compression
stockings (GCS), and intermittent pneumatic
compression (IPC)) should be avoided.
(3) Prophylaxis should be instituted as soon as
feasible after primary hemostasis has been ensured.
The combination of IPC and either LDUH or
low-molecular-weight heparin (LMWH) should be used.
(4) In patients who have an incomplete SCI and
evidence of a perispinal hematoma on computed
tomographic (CT) scan or magnetic resonance imaging
(MRI), the administration of LMWH should be delayed
for 1 to 3 days.
(5) IPC and/or GCS should be used when prophylaxis
with an anticoagulant is contraindicated.
(6) An inferior vena caval filter should not be used
as primary thromboprophylaxis against PE, but should
be used when anticoagulation is contraindicated or
after a documented PE in a patient already receiving
anticoagulant therapy.
(7) During the rehabilitation phase after acute SCI,
either LMWH prophylaxis should be continued or the
patient should be converted to an oral vitamin K
antagonist to achieve an international normalized
ratio (INR) of 2 to 3.
(8) DVT prophylaxis should be continued for 3
months.
Neurologic examination. The neurologic examination
determines areas that need radiologic evaluation and
establishes a baseline for subsequent assessment.
The following neurologic examination can be
performed quickly and efficiently. A more thorough
examination can be performed if indicated.
a. Consciousness. Is the patient alert, oriented, and
responsive?
b. Motor system. Function and strength of the major
muscle groups are graded with reference to the
normal segmental innervation. If possible,
cerebellar function can be assessed by having the
patient touch finger to nose.
c. Sensory system. This examination includes an
assessment of proprioception and the patient's
response to light touch and pinprick. Perirectal
sensation and the presence of either the bulbocavernosus reflex or the anal-cutaneous reflex
are important indicators of the preservation of
distal function (sacral sparing), which can mean a
more favorable prognosis. A rectal examination is
performed to assess voluntary contraction of the
anal sphincter. The most caudad level with normal
motor and sensory function is designated as the
level of injury (Table-5).
d. Cranial nerves. The specific nerves evaluated depend
on the patient's level of injury, associated injury,
and level of consciousness. The responses most often
evaluated include the pupillary reflex, ocular
movement, tongue movement, and function of the
trigeminal and facial nerves.
e. Reflexes. Commonly tested reflexes include the
biceps, triceps, patellar, Achilles, abdominal, cremasteric, and Babinski.
Radiologic evaluation
a.
The incidence of cervical spinal injury in comatose
trauma patients is 7%. Therefore, radiologic
assessment of the spine is essential after traumatic
injuries, particularly in those individuals who are
either comatose or have signs and symptoms referable
to a SCI.
b.
Assessment begins with plain X-ray films. The
three-view cervical spine series, composed of
lateral, anteroposterior, and open-mouth odontoid
views, detect most cervical spinal injuries. For
C-spine injuries, a useful method of analysis of the
plain films addresses the following:
(1) Adequacy and quality of the occipital-cervical
junction (C1-2), C3-7, and the C7-T1 junction.
(2) Alignment of the anterior edge of the vertebral
body, the posterior edge of the vertebral body, the
spinolaminar junction, and the tips of the spinous
process (Figure-4).
(3) Bones should be assessed for fractures of the
vertebral body, pedicle, lamina, and spinous
process.
(4) Cartilage assessment includes analysis of the
disk space and the facet joints.
(5) Soft tissue space should be observed for edema
and other abnormalities.
As many as 15% to 20% of patients with a C-spine
injury do not have an abnormality on
the plain film. A more comprehensive radiologic
evaluation can be indicated.
Table-5. Muscle group with
corresponding level of innervation |
Injury Level |
Sensory Deficit |
Affected Muscle
Group |
C4 |
Acromioclavicular
joint |
Diaphragm |
C5 |
Antecubital fossa
(lateral) |
Shoulder rotators
and abductors, elbow flexors |
C6 |
Thumb |
Supinators,
pronators, wrist extensors |
C7 |
Middle finger |
Elbow extensors,
wrist flexors |
C8 |
Little finger |
Finger flexors,
distal phalanx |
T1 |
Antecubital fossa
(medial) |
Intrinsic hand
muscles |
L2 |
Upper anterior
thigh |
Hip flexors |
L3 |
Medial femoral
condyle |
Knee extensors |
L4 |
Medial malleolus |
Ankle dorsiflexors |
L5 |
Dorsum of foot |
Toe extensors |
S1 |
Lateral heel |
Plantar flexors |
S2-5 |
Popliteal fossa,
ischial tuberosity, perianal area |
Sphincter ani,
bulbocavernosus reflex |
Figure-4. The lateral cervical spine. Spinal
column stability after traumatic injury is often
based on a system that divides the spine into four
longitudinal lines from anterior to posterior,
starting with the anterior edge of the vertebral
body (AV), the posterior edge of the vertebral body
(PV), the spinolaminar junction (SL), and the tips
of the spinous process (SP). Each line provides the
border for three columns (I, II, and III).
Disruption of two or more of these columns indicates
spinal instability. |
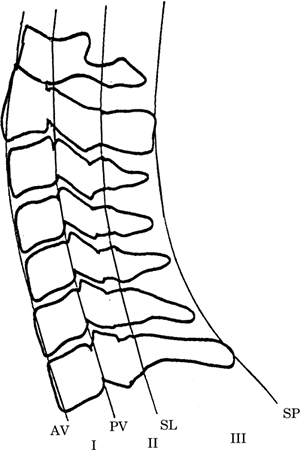 |
(1) CT scan is helpful to do the following:
(a) Define bony and soft tissue abnormalities
(b) Evaluate the lower C-spine
(c) Measure spinal canal and neuroforaminal diameter
(d) Provide detailed anatomy of the facet joints
(e) Detect hematoma formation
(f) Determine compression of the spinal canal and
spinal stability
(g) Detect a unilateral jumped facet or bone
fragments in the canal or root foramen
(h) Confirm complete reduction of a dislocation
(i) Demonstrate hyperdense acute blood or
retropulsed vertebral body fragments
(2) Axial CT scan through C1 and C2 and through the
lower C-spine is indicated in situations in which
poorly visualized or suspicious areas are noted on
plain films. The negative predictive value of the
combination of a normal three-view cervical spine
series supplemented with CT scan through areas that
are poorly visualized and "suspicious" is 98% to
100%.
(3) MRI is indicated within 48 hours of injury for
symptomatic SCI and in comatose trauma patients who
can have a cervical spinal injury. MRI is helpful
for the following:
(a) Determining the degree of injury to the soft
tissue contents of the spinal canal
(b) Identifying ligamentous injury, nerve root
compression, and pathologic signals from the spinal
cord itself
(c) Predicting functional neurologic outcome in the
subacute stage of SCI
(d) Visualizing the epidural and subarachnoid spaces
(e) Detecting elevation of the anterior or posterior
longitudinal ligaments
(4) Flexion-extension films performed under
fluoroscopic guidance are recommended as an
alternative to MRI to evaluate the degree of
ligamentous stability in awake patients who have
symptoms of cervical spinal injury and in comatose
trauma patients.
Neuroprotective strategies
a. Spinal alignment
(1) Studies have shown that movement of the injured
segment results in exacerbation of the initial
injury. Therefore, an important neuroprotective
strategy is to relieve cord compression and ischemia
and prevent further neurologic compromise by
immediate and effective immobilization of the spine
with either tongs or halo traction devices. Failure
to accomplish this can lead to either loss of
residual neurologic function or even ascension of
the patient's level of neurologic injury.
(2) Patients who have unstable injuries are placed
in traction to align and immobilize
the spine, decompress neural structures, and prevent
further injury. Diminished pressure on the cord
improves microvascular circulation, which could
reduce spinal cord edema. If there is no bony
instability, traction is not usually needed for
patients who have sustained penetrating injuries.
(3) Thoracic and lumbar fractures and dislocations
can initially be stabilized by restricting the
patient to bed rest and turning him or her in a
log-roll fashion to preserve spinal alignment.
Surgical intervention usually stabilizes
subluxations in these regions.
b. Surgical reduction and stabilization could be
necessary for dislocations that cannot be reduced by
traction and/or manipulation because of the nature
of the injury. Surgical decompression within the
first 2 hours of injury can increase the chances of
recovery. The decision as to the timing of surgery
after spinal injury depends on these factors:
(1) Assessment of the underlying injury
(2) Failure of medical, manipulative, and bracing
procedures to achieve adequate alignment
(3) The documented benefit of early decompression or
stabilization of the spine
(4) The presence of progressive neurologic deficits
and/or refractory pain
(5) The severity of any coexisting illnesses,
infections, or trauma
(6) The documented benefit of earlier patient
mobilization and rehabilitation from early surgical
intervention (within 8 to 72 hours after injury)
c. Physiologic therapy
(1) Cooling has been shown to be effective in the
treatment of SCI. The limitations of the clinical
studies are small cohorts and lack of controls. The
high mortality is also of concern. The effect of
modest hypothermia on spinal recovery after trauma
continues to be evaluated, but clinical efficacy in
human SCI has demonstrated little benefit thus far.
Conversely, hyperthermia is deleterious. Aggressive
measures to prevent hyperthermia should be taken to
minimize the propagation of secondary neuronal
damage after traumatic SCI.
(2) Some advocate hypertension to improve perfusion
in the posttraumatic patient who has evidence of
impaired
autoregulation and hypoperfusion of the spinal cord.
Definitive human data are not available. The MAP is
maintained in the normal to high-normal range (85 mm
Hg) by either volume replacement (nondextrose-containing
crystalloid, colloid, or blood products) if
hemorrhagic shock predominates or inotropes and
vasopressors if neurologic shock is the cause of
hypotension. More aggressive achievement of
hypertension carries the risk of intramedullary
hemorrhage and edema.
(3) Glucose-containing solutions are avoided.
Studies in experimental models have shown that even
minimally increased blood glucose levels worsen
neurologic outcome.
d. Pharmacologic therapy
(1) Corticosteroids administered after acute SCI are
currently considered the standard of care. Previous
studies have shown that steroids have the potential
to stabilize membrane structures, maintain the
blood-spinal cord barrier, enhance SCBF, alter
electrolyte concentrations at the site of injury,
inhibit endorphin release, scavenge damaging free
radicals, and limit the inflammatory response after
injury.
The current use and dosage of corticosteroids are
based on the National Acute Spinal Cord Injury II
Study (NASCIS) reported in 1990. This prospective,
randomized, placebo-controlled study compared
high-dose methylprednisolone (MP) with naloxone and
placebo in patients who had complete and incomplete
acute SCI. MP, administered within 8 hours of injury
as a 30 mg/kg bolus over the first hour and then
infused at 5.4 mg/kg/hour for 23 hours, was
associated with improvement in motor function and in
sensation at 6 months of follow-up as compared to
both naloxone and placebo.
The National Acute Spinal Cord Injury III Study,
published in 1997, showed that if MP is given within
3 hours of SCI, the steroid infusion need be
continued for only 24 hours. If therapy is initiated
within 3 and 8 hours of injury, however, the steroid
infusion should be administered for 48 hours. Both
studies demonstrated an increase in medical
complications in steroid-treated patients: longer
hospitalizations, increased wound
infections, and an increased incidence of pneumonia,
GI bleeding, and severe sepsis.
(2) Mannitol, 0.25 to 1 g/kg, can be used to treat
cord edema. The ensuing osmotic diuresis
necessitates close attention to the patient's
intravascular volume.
(3) Hypertonic saline (HS) use in experimental
models of acute SCI has suggested that it can
enhance the delivery of MP and prevent
immunosuppression, leading to improvements in
overall neurologic function and survival rates after
SCI. No conclusive evidence has yet been published
demonstrating similar benefits in human SCI. The use
of HS in the setting of acute SCI, therefore,
remains largely experimental.
(4) Other pharmacologic agents have been studied in
acute SCI as treatment modalities either to limit
secondary injury or enhance neurologic recovery.
Such agents have included GM1 ganglioside,
tirilazad, naloxone, thyrotropin-releasing hormone,
and nimodipine. None of these agents has clearly
demonstrated an improvement in outcome after acute
SCI, and their use is therefore not currently
recommended.
Anesthetic management of acute SCI
Preoperative evaluation. The SCI patient frequently
has multiple medical complications that can impact
the anesthetic plan.
a. Indicated studies include complete blood count,
serum electrolytes, blood urea nitrogen, creatinine,
glucose, liver function tests, and a urinalysis. A
preoperative electrocardiogram (ECG), arterial blood
gas, chest x-ray, and pulmonary function tests can
also be indicated.
b. Airway evaluation is necessary. Examination of the
airway must include the oropharynx with a Mallampati
classification and range of motion of the neck with
particular attention to any limitation from either
pain or neurologic symptoms. If movement elicits any
abnormality, the offending position is avoided.
Airway problems are most frequently encountered in
patients who have atlantoaxial subluxations,
traumatic C-spine injuries in combination with
facial trauma, severe kyphoscoliosis or spinal
deformities, and spinal stabilization devices. When
the patient is in a halo brace or other cervical
fixation device, plans for either an awake tracheal
intubation or other technique to secure the airway
should be made.
c. Neurologic evaluation is performed preoperatively to
document any preexisting neurologic deficits.
Regional anesthesia is chosen only after careful
evaluation and review of all preexisting deficits.
d. Pulmonary evaluation must consider the level of SCI.
For instance, patients who have a C-spine injury
have restrictive pulmonary defects and marked
reductions in lung volumes that predispose them to
hypoxemia. Injuries of the cervical and high
thoracic spine engender difficulties with clearance
of secretions, which could also predispose patients
to hypoxemia and hypercarbia.
e. Cardiac evaluation is essential to elicit evidence
of cardiovascular dysfunction from either the acute
SCI or preexisting abnormalities. In addition, an
assessment of the degree of orthostatic hypotension
and the risk of autonomic hyperreflexia should be
made.
Monitoring. Decisions regarding the utilization of
advanced monitoring are based on the level of injury
and neurologic deficit, the complexity and length of
the surgical procedure, and any preexisting
underlying medical diseases.
a. Neurophysiologic monitoring
is often indicated for patients who have no
neurologic injuries but are at high risk owing to
the instability of their spinal abnormalities and
for patients who have incomplete neurologic injuries
and are undergoing operations for spinal
stabilization. Neurophysiologic monitoring could
consist of an intraoperative wake-up test,
somatosensory evoked potential (SSEP) monitoring, or
motor evoked potential monitoring. SSEPs monitor the
posterior columns of the spinal cord, whereas motor
evoked potentials monitor the anterior portion of
the spinal cord. For a more comprehensive
description of spinal cord monitoring.
b. Intracranial pressure monitoring can be necessary
for patients who also have head injuries.
c. Routine monitors (ECG, pulse oximetry, capnography,
noninvasive BP, temperature) are employed for every
procedure. A latex-free urinary catheter is used to
monitor the patient's volume status.
d.
In patients who have spinal shock, the institution
of direct BP monitoring is indicated, ideally before
induction of anesthesia. The arterial catheter is
also useful for blood gas measurements and other
laboratory determinations that can be necessary
intraoperatively.
e.
Early use of pulmonary artery catheters during
spinal shock is appropriate. Measurement of
intracardiac pressures (central venous pressure
[CVP], pulmonary capillary wedge pressure [PCWP],
left ventricular end diastolic pressure [LVEDP]) in
conjunction with cardiac output and BP is necessary
to differentiate hypovolemia from low systemic
vascular resistance (SVR). The information obtained
is helpful in determining the appropriate form of
management (fluid versus vasopressors) and in
monitoring the response to therapy.
(1) Patients who have low SVR can be treated with
titrated infusions of a direct-acting alpha-agonist,
as above.
(2) Patients who are hypovolemic can receive fluid
boluses of 250 to 500 mL. A Starling curve,
identifying the optimal fluid filling pressure, can
be derived from the changes in intracardiac
pressures (CVP, PCWP, LVEDP), cardiac output, and BP
in response to the fluids.
Anesthetic technique
Securing the airway without causing or exacerbating
SCI is the principal concern.
(1) An awake intubation has the advantage that the
patient acts as a monitor to avoid worsening the
SCI. In addition, a neurologic evaluation can
document the absence of any new changes. An awake
intubation also avoids the use of succinylcholine
and the attendant risk of hyperkalemia.
(2) A blind nasal endotracheal intubation is often
recommended as one of the best means to avoid spinal
manipulation during intubation. This approach is
contraindicated with facial trauma or a basilar
skull fracture. Topical application of 0.2%
phenylephrine hydrochloride in 4% lidocaine is
essential to shrink nasal tissues and limit
bleeding. Anesthesia of the tongue can be achieved
using 2% lidocaine ointment or local anesthetic
sprays. Anesthesia of the vocal cords and larynx is
achieved by using the combination of a transtracheal
injection of 4% lidocaine via a percutaneous
puncture of the cricothyroid membrane and superior
laryngeal nerve blocks with the bilateral injection
of lidocaine 1%, 2 mL, into the thyrohyoid membrane
just above the lateral wings of the thyroid
cartilage. An alternative technique uses nebulized
lidocaine 4% to a maximum dose of no >4 to 5 mg/kg.
Calculations of toxicity must include all topical
and injected local anesthetics.
(3) Fiberoptic endotracheal intubation can be used
in a nonemergent situation after anesthetizing the
airway as detailed in the preceding text. With the
oral route, the use of either a bite block or large
oral airway containing a central passageway for the
fiberoptic scope is essential. In addition, oral
intubation often mandates the use of mild sedation
to facilitate patient acceptance. Blood, debris, and
vomitus in the airway mitigate against fiberoptic
intubation.
(4) Direct laryngoscopy can be appropriate if the
previous methods do not seem feasible. Neutrality of
the neck must be maintained to prevent further SCI.
Extension or flexion during intubation can cause
displacement and worsening of the original SCI.
In-line manual cervical immobilization appears to be
the safest method to minimize spinal column motion.
(5) When either severe facial trauma or neck
instability exists or the airway is lost, a surgical
airway via cricothyrotomy or tracheotomy can be
necessary. The method selected for intubation
depends on perceived airway difficulties, coexisting
disease and trauma, and other factors including
facial trauma and soft tissue swelling.
Induction
(1) The sympathetic function of SCI patients is
unpredictable. Because they are also frequently
hypovolemic, the induction should proceed slowly in
an elective situation. When the patient has a full
stomach, a rapid-sequence induction is indicated
after adequate volume replacement has been
accomplished.
(a) Ketamine, 1 to 2 mg/kg i.v., provides a more
stable hemodynamic profile during induction. It has
the added advantage of amplifying the
electrophysiologic monitor's signal amplitude, but
its use is not generally recommended in the presence
of intracranial hypertension.
(b) Etomidate provides cardiovascular stability
during induction.
(2) During the induction of general anesthesia, the
maintenance of a spinal cord perfusion pressure of
at least 60 mm Hg (ideally 80 to 90 mm Hg) is
essential. The
patient in the early phase of spinal shock after a
high cord injury is at risk of developing
bradycardia or asystole. Accordingly, some have
advocated the use of a prophylactic anticholinergic
to avoid this complication.
(3) Excessive fluctuations of cardiovascular
parameters should be treated with direct-acting
agonists and antagonists. These drugs should
preferably be short acting and delivered via
intravenous infusion. Sympathetic agonists and
antagonists that release catecholamines indirectly
are avoided.
Muscle relaxants. The SCI patient whose injury
involves skeletal muscles develops a supersensitivity to depolarizing muscle relaxants.
With muscle denervation, the number of postsynaptic
acetylcholine receptors increases greatly and
amplifies any small neuromuscular signal that can be
present. When depolarized by succinylcholine, pores
on the neuromuscular junction open maximally,
allowing massive egress of stored intracellular
potassium. The occurrence of succinylcholine-induced
ventricular fibrillation secondary to this acute
hyperkalemic response has been reported.
Because the time course for the development of the
extrajunctional receptors is not known precisely
(but can be as short as 24 hours), succinylcholine
is best avoided even in recently injured patients.
It is also important to realize that the magnitude
of the potassium release is more a function of the
amount of muscle mass affected than of the dose of
depolarizing drug given. Even small doses of
succinylcholine have triggered significant
hyperkalemia. The administration of succinylcholine
should be avoided in patients who have SCI.
Positioning. Spinal operations are most often
performed in the prone position. Patients can be
anesthetized on the bed or stretcher and then log
rolled onto the operating table. Important goals
include maintaining the head and neck in a neutral
position; providing adequate padding to the chest,
abdomen, head, and extremities; and avoiding
excessive neck flexion or extension. Special
attention should be given to the endotracheal tube
because significant movement or obstruction can
occur with changes of position. Sudden changes of
position should also be avoided because they can
have significant hemodynamic consequences owing to
the lack of adequate compensatory
vasoconstrictor and cardiac reflexes to maintain
venous return and cardiac output.
The head is positioned so that the bony prominences
support its weight without pressure on the eyes,
ears, or nose. The position of the head can be
adjusted slightly every 15 minutes or so throughout
the procedure to ensure adequate perfusion under the
weight-bearing area.
When an awake intubation is employed, the use of
nerve blocks instead of sedatives enables the
patients to position themselves. This allows a
neurologic examination to be performed after
positioning and helps ensure that the operative
position does not aggravate the injury. Information
gleaned from the preoperative neurologic examination
(range of motion, motions that worsen symptoms) also
helps to prevent the positioning from exacerbating
the neurologic injury.
Maintenance. The choice of anesthetic drugs is
guided by the patient's underlying condition. The
anesthetic drugs selected should maintain optimal
SCBF. The use of neurologic monitors necessitates
the avoidance of drugs (e.g., volatile anesthetics)
that either suppress the monitored responses or
cause fluctuations in the anesthetic depth, which
can confuse the interpretation of the evoked
responses.
In general, drug regimens that utilize opiate
infusions serve this purpose. Regional techniques
can be considered, but the high incidence of
coexisting injury and the frequency of hemodynamic
and respiratory compromise virtually guarantee that
general anesthesia is preferable.
Some evidence indicates that hypocapnia decreases
SCI from ischemia. However, because hypocapnia could
also compromise perfusion, normocapnia is
recommended.
Fluid management. Fluid administration is based on
the estimated preoperative fluid deficits,
intraoperative blood and fluid losses, and a
knowledge of the effect of the level of SCI on
cardiac and pulmonary function. Meticulous fluid
management is essential because patients who have
high thoracic and cervical spine injuries have an
increased propensity for developing pulmonary edema.
In addition, cervical spine injury can cause cardiac
dysfunction with decreased inotropy and chronotropy
(from reduced sympathetic neural input to the
heart). Whether to use either crystalloid or colloid
for volume resuscitation is of less importance than
the need to avoid glucose-containing solutions,
which are known to exacerbate SCI.
Temperature regulation. SCI patients have impaired
thermoregulation below the level of the injury.
Prophylactic measures to prevent intraoperative
hypothermia should be aggressively instituted. These
include a warm ambient environment, warming of
intravenous fluids, standard warming mattresses,
humidification of the respiratory circuit, and
forced hot-air blankets. Keep in mind, however, that
hyperthermia exacerbates neurologic injury.
Postoperative care. Even patients who had adequate
respiratory function before surgery could require a
weaning period postoperatively before extubation
owing to the residual effects of the anesthetics.
Because of the potential difficulty in reintubating
the trachea of the SCI patient, determination of the
appropriate time for extubation should be extremely
conservative. Critical care weaning parameters are
used in place of routine extubation criteria after
most operative procedures. Once the trachea has been
extubated, the patient is monitored for several
hours to ensure that the respiratory status does not
deteriorate.
Extubation criteria include the following:
a. Blood gases
(1) pH >7.3
(2) Pao2 >60 mm Hg
(3) Paco2 <50 mm Hg
(4) Ratio of partial pressure of arterial oxygen to
inspired fraction of oxygen (Pao2/fraction of
inspired oxygen [Fio2]) >200
b. Pulmonary functions
(1) Maximal negative inspiratory forces <-25 cm H2O
(2) VC >15 mL/kg
(3) Respiratory rate <25/minute
(4) Dead space-to-tidal volume ratio <0.6
c. Other
(1) Patient conscious and oriented
(2) Unlabored, or minimally labored, breathing
(3) Ability to generate a cough
(4) Minimal volume of tracheal secretions
(5) Stable cardiac function
(6) Optimal intravascular fluid volume and
electrolyte status
(7) Absence of infection
Chronic SCI. As improved emergency medical care
increases the survival of patients who have
high-level spinal cord injuries, one is more likely
to encounter patients who now have chronic SCIs
scheduled for surgical procedures for spinal and
other indications. Many issues raised regarding
acute SCIs apply equally to patients who have
chronic injury. There are, however, a number of
additional medical problems (Table-6).
Table-6. Medical problems of the patient who has
chronic spinal cord injury
|
System |
Abnormality |
Relevant Comment |
Cardiovascular |
Autonomic hyperreflexia
↓Blood volume
Orthostatic hypotension |
Susceptible to hypertensive crisis if SCI level is
above T6. Positional changes and intrathoracic
pressure can cause hypotension. |
Respiratory |
Muscle weakness
↓Respiratory drive
↓Cough |
SCI patient is susceptible to postoperative
pneumonia and can be difficult to wean from
mechanical ventilation. |
Muscular |
Proliferation of acetylcholine receptors
Spasticity |
Hyperkalemia from succinylcholine. |
Genitourinary |
Recurrent urinary tract infections
Altered bladder emptying |
Can lead to renal insufficiency, pyelonephritis,
sepsis, or amyloidosis. Frequent catheterization can
cause latex allergy. |
Gastrointestinal |
Gastroparesis
Ileus |
Susceptible to aspiration. |
Immunologic |
Urinary tract infection
Pneumonia
Decubitus ulcers |
Watch for subtle signs of infection and sepsis.
Questionable risk of seeding an infection from
invasive monitoring. |
Skin |
Decubitus ulcers |
Prevention. |
Hematologic |
Anemia
Risk of DVT |
DVT prophylaxis. |
Bone |
↓Bone density |
Osteoporosis, hypercalcemia, heterotopic
ossification, muscle calcification, pathologic
fractures. |
Nervous system |
Chronic pain |
Perioperative pain can be difficult to control.
|
SCI, spinal cord injury, DVT, deep vein thrombosis.
|
Preoperative. Respiratory complications are the most
common cause of morbidity in SCI patients. Pneumonia
is second only to anoxia at the time of injury as
the cause of death. Any fever with a leukocytosis or
physical or radiographic evidence of pneumonitis
should be treated aggressively.
Patients who have chronic injury are more prone to
episodes of hypertension secondary to autonomic
hyperreflexia, especially with lesions above T6.
Muscle spasms also occur because of hyperactive
spinal reflexes without the modulating effect of
cortical, brain stem, and cerebellar centers. This "mass reflex" could require the therapeutic use
of skeletal muscle relaxants in the awake patient.
Intraoperative
Regional anesthesia can offer some advantage for
patients who still have instability of the cervical
spine or whose initial repair limits their cervical
range of motion. Should general anesthesia prove
necessary for this subset of patients, all
precautions for airway manipulation should be
employed.
General anesthesia and regional anesthesia are
equally effective in preventing autonomic
hyperreflexia. Techniques based on the use of
oxygen/nitrous oxide/narcotics seem to be less
efficacious in this regard. When deciding on the
appropriateness of a regional technique, one should
consider the site of injury as well as the site of
surgery. The patient's underlying hemodynamic status
should also be considered. Regardless of the
technique, direct-acting vasodilators (e.g.,
nitroprusside), alpha-adrenergic blocking drugs
(e.g., phentolamine), antiarrhythmics (e.g.,
lidocaine, esmolol), atropine, and other
antihypertensives (e.g., labetalol) should be
readily available.
Chronic SCI puts all patients at risk for a
hyperkalemic response to depolarizing muscle
relaxants. Decreasing the dose does not reliably
prevent the response. Therefore, succinylcholine is
to be avoided.
As the receptors at the neuromuscular junction of
denervated muscle are upregulated, basing the dose
of nondepolarizing muscle relaxants on twitch
response from a denervated limb leads to a relative
overdose of muscle relaxant. This should be
considered when giving the initial dose, when
reversing the relaxation at the end of the
procedure, and when judging readiness for
extubation.
Urinary retention and urinary tract infections are
persistent problems. A distended bladder can trigger
the hypertensive response of autonomic
hyperreflexia. Therefore, in addition to
routine monitors, these patients should have a
latex-free urinary catheter inserted for most
procedures.
Postoperative. Postoperative management, including
when to extubate the trachea, should be guided
primarily by the requirements of the surgical
procedure and the patient's underlying medical
condition.
III. Spinal cord tumors
Spinal tumors can be primary or metastatic in
origin. The medical problems associated with the
patient are determined by the location of the spinal
lesion and by the location of the primary tumor in
metastatic lesions. Depending on the length of time
since the presentation of the spinal lesion and its
associated signs and symptoms, these patients could
have many of the same complications seen in patients
after traumatic injury to the spinal cord.
Preoperative management
Lesions of the upper spinal cord are the most
damaging physiologically. Respiratory impairment
secondary to loss of activity of the intercostal
muscles and/or diaphragmatic is common. A blood gas
measurement is indicated to determine the patient's
baseline respiratory status. In addition, the
decreased ability to clear secretions can frequently
result in pneumonitis, which can require further
intervention. Such infections are not always
associated with elevated temperature, nor does
elevated temperature always point to an infection
because temperature regulation is frequently
impaired as well.
Cardiovascular tone could be diminished with
resulting hypotension. This also occurs in patients
with acute SCI. One must differentiate neurogenic
hypotension from the hypotension resulting from
dehydration and malnutrition, commonly associated
with patients who have cancer. Such dehydration
could be exacerbated in patients who have recently
undergone diagnostic radiographic tests that
involved the use of contrast dyes because these
usually act as diuretics.
In addition to the basic chemistries, preoperative
blood determinations should include liver function
tests to identify the presence, if any, of
metastasis to the liver. The possibility of
pituitary and adrenal insufficiency should also be
considered because these are frequent sites of
metastatic lesions.
Premedication should be kept to a minimum because it
is useful to be able to reassess neurologic function
immediately before operation. In particular,
sedatives are avoided in situations in which the
respiratory status is already impaired.
Intraoperative management
The planned procedure and the location of the
lesion dictate the choice of monitors beyond the
routine. Patients who exhibit signs of spinal shock
are managed more effectively with the cardiovascular
information provided by a pulmonary artery catheter
and an arterial line. Those patients at risk for
autonomic hyperreflexia can also require the "beat-to-beat" BP monitoring provided by an
arterial line.
The positioning of these patients for the procedures
frequently places the surgical site above the level
of the heart. This increases the risk for pulmonary
air embolism. The anesthetic plan should incorporate
a monitor for the detection of air embolism (e.g.,
precordial Doppler, transesophageal
echocardiography, pulmonary artery pressure) as well
as an appropriately positioned CVP line to aspirate
air.
The risk of hyperkalemia with the use of
succinylcholine is increased as it was for the
patient who has SCI. The use of nondepolarizing
relaxants is recommended to ensure a quiet surgical
field. However, because of upregulation of receptors
in the neuromuscular junction secondary to
denervation, care should be taken to select an
appropriate site for monitoring twitch suppression
via the nerve stimulator.
Maintenance of anesthesia can be accomplished with
any general anesthetic technique. However, in
procedures in which the use of SSEPs is anticipated,
drugs that suppress the monitored potentials are
avoided. In such circumstances, a nitrous
oxide/oxygen/narcotic technique is more appropriate.
Frequent changes of anesthetic depth are avoided
because they interfere with the interpretation of
the SSEPs.
Postoperative management. The decision regarding
early extubation of these patients is made on a
case-by-case basis. Early extubation in a fully
awake patient facilitates postoperative neurologic
examination. The appropriateness of early extubation
depends on the patient's baseline respiratory
function, location and size of the tumor, and
duration and degree of difficulty of surgical
dissection. Patients who were operated for
high-level lesions require close monitoring for as
long as 48 to 72 hours postoperatively. During this
time, edema at the surgical site can cause renewed
compression of the spinal cord and lead to
progressive respiratory compromise and loss of
protective gag reflexes. Reintubation, when
necessary, should be done early in the course of
respiratory deterioration. This allows accomplishing
control of the airway in a patient who has a
potentially unstable neck in as nonemergent an
environment as possible.
IV. Scoliosis
General
Scoliosis is a structural disease of the vertebral
column causing lateral curvature of the spine with
rotation of the vertebra.
Scoliosis most frequently involves the thoracic and
lumbar regions.
The severity of the disease is expressed in "degrees of lateral angulation." A larger degree
of angulation represents more severe disease. The
side of the convexity of the curve designates it as
a right or left curvature.
Idiopathic scoliosis, accounting for almost 75% of
cases, occurs most frequently in adolescent girls.
Other types are classified as nonstructural (caused
by gait/posture), congenital, neuromuscular, and
traumatic.
The degree of severity of scoliosis correlates
directly with the degree of respiratory dysfunction.
Curvatures of <60° are not usually associated with
significant pulmonary involvement, whereas patients
who have curvatures of >100° are typically severely
impaired.
Pulmonary function testing typically reveals a
restrictive pattern owing to the reduction in chest
wall compliance with reductions in all measures of
lung capacity and volume. The greatest decrease is
noted in the VC. Blood gas analysis reveals a lower
Pao2, which is primarily because of the
ventilation-perfusion mismatch that occurs. Paco2 is
not usually altered unless an obstructive pulmonary
component has also developed, a condition more
common in the elderly.
In patients who have more severe thoracic disease,
rib cage deformities lead to the underdevelopment of
the pulmonary vasculature. This is exacerbated by
hypoxic pulmonary vasoconstriction and can result in
potentially significant elevations in pulmonary
vascular resistance. Right ventricular hypertrophy
and right atrial enlargement can occur as a result
of these changes in pulmonary vascular resistance.
Eventually patients develop irreversible pulmonary
hypertension and cor pulmonale.
Preoperative management
The preoperative assessment should include
information about the location and degree of
scoliosis as well as its etiology. Both location and
degree of disease play a role in determining the
amount of pulmonary/cardiac involvement one can
expect to find. Scoliosis owing to concurrent
neurologic motor deficits can alter the choice of
muscle relaxants.
Physical examination indicates necessary testing.
Patients who have limited exercise tolerance
require further pulmonary function testing to
ascertain the extent of their pulmonary
insufficiency. Patients who have vital capacities
below 25 mL/kg are high operative risks.
Preoperative sedation is avoided in patients who
have significant pulmonary compromise.
Intraoperative management
Decisions regarding monitoring are guided by the
extent of pulmonary and cardiac involvement. One
should consider an arterial line for blood gas
measurement in any patient who has even moderately
reduced pulmonary reserve. An arterial line is also
necessary for closer monitoring of BP when "deliberate hypotension" is employed to reduce
intraoperative blood loss.
Pulmonary artery catheter monitoring should be
reserved for those patients who have either
pulmonary hypertension or significantly diminished
right ventricular output.
No advantage can be demonstrated for any particular
anesthetic induction or maintenance regimen. The
underlying hemodynamic condition of the patient
should be used to guide the choice and dose of
drugs.
Placement of spinal instrumentation involves
traction to correct the curvature. SSEPs are
frequently utilized to monitor the sensory component
of the spinal cord. However, SSEPs monitor only the
posterior cord. Anterior cord elements could be
damaged despite normal SSEPs. When SSEP monitoring
is utilized, one should limit either the use or the
concentration of inhalation anesthetics, which
interferes with signal interpretation (see preceding
text).
A wake-up test definitively confirms adequate blood
flow to, and therefore the function of, the anterior
motor portion of the spinal cord. This is
accomplished by having the patient awaken and move
the lower extremities in response to verbal command.
With a multitude of short-acting muscle relaxants
and maintenance anesthetics, it has become
relatively simple to maintain a deep level of
anesthesia until the time of the wake-up test yet to
allow for a quick awakening at the appropriate time
during the procedure.
Postoperative management. The spinal fusion and rodding procedure prevents further deterioration of
pulmonary and cardiac parameters. These
abnormalities are not typically corrected by the
procedure. Careful consideration of the patient's
baseline status helps to determine which patients
are suitable candidates for early extubation.
|